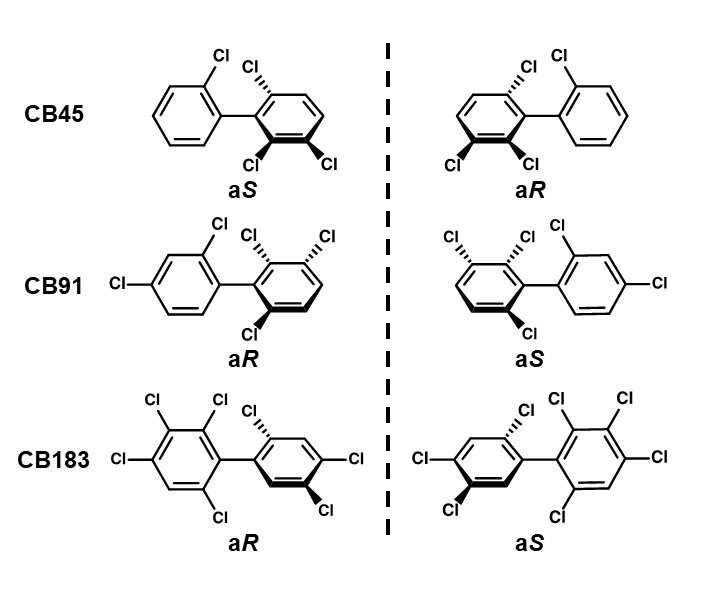
Polychlorinated biphenyls (PCBs) have been widely used in industrial and commercial products including plastics, paints, electronic equipment and insulating fluids. Their manufacture was extensively banned from the late 1970s onwards due to their toxicity, but large amounts still remain in our environment and accumulate inside animals’ bodies.
Chiral PCBs are PCBs that have two mirror-image isomers; these isomers are identical reflections of each other with the same composition. Chiral PCBs are particularly dangerous because they have more chlorine atoms, which are hard for the body to break down, so they can accumulate in the body easily and their isomers are metabolized differently, causing isomer-specific toxicity (particularly neurodevelopmental issues). However, the process behind this selective metabolism was not previously known.
To address this, a research group has illuminated how enzymes produced by the body unevenly metabolize the mirror-image isomers. These results will make it possible to estimate PCB metabolism and detoxification pathways in animals. They will also contribute towards the development of technology to make predictions about chiral PCBs’ mirror isomers, so that we can obtain a better understanding of potential toxicity in humans and other mammals.
The research results were published in Environmental Science & Technology and Chemosphere.
Even though the manufacture and use of PCBs was banned around 50 years ago, they still remain in the environment. It has been discovered that PCBs accumulate inside the bodies of humans and other animals through food consumption. In particular, PCBs with many chlorine bonds are water-resistant and do not break down easily.
This enables high concentrations of these PCBs to accumulate inside animals’ bodies, which adversely affects their health. PCBs’ toxicity is induced by the aryl hydrocarbon receptor (AhR), causing similar adverse effects to dioxin poisoning such as cancer, teratogenesis and immune system damage. Research is being conducted on the particular types of PCB widely known to cause these effects, which are dioxin-like PCBs with one ortho chlorine substitution in the biphenyl ring of their chemical structure, or PCBs with no substitutions.
However, if a PCB has more than three chlorine substitutions at the ortho position of the biphenyl ring, it becomes a mirror-image isomer called chiral PCB. These chiral PCBs do not demonstrate dioxin-like toxicity, but are far more dangerous, binding with the ryanodine receptors (RyR) in organisms to become neurotoxic.
The two mirror-image isomers (called atropisomers) in chiral PCBs have identical physical and chemical properties and exist at a 1:1 ratio in commercial chiral PCBs, but biased ratios are often observed in the environment and in animals such as earthworms and whales, as well as humans. It is believed that this kind of unbalanced ratio is mainly caused by metabolism and that one of a chiral PCB’s atropisomers is more affected by the metabolic reaction, thus reducing its concentration.
Until now, very little research has been carried out into differences in how these atropisomers are metabolized nor the structural arrangement of the metabolic enzymes.
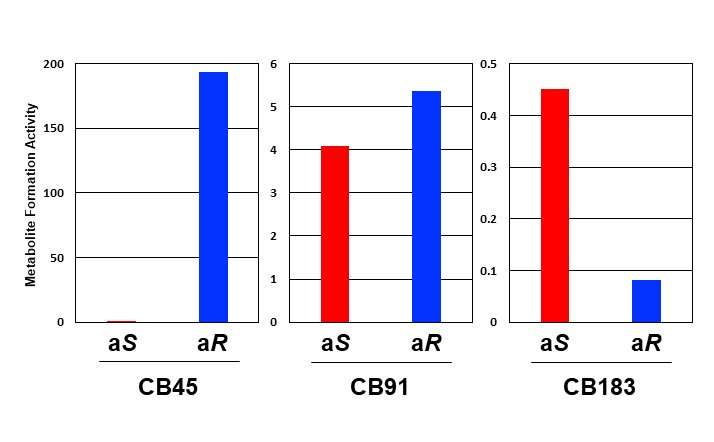
To address this knowledge gap, the team conducted research focusing on the metabolic enzyme cytochrome P450 (CYP enzyme). The CYP enzyme reacts with foreign compounds that enter an animal’s body (for example, chemicals or pollutants in food or medicines). CYP can convert them into water-soluble compounds and promote their expulsion from the body. Previous research by this group has shown that CYP enzymes hydroxylate and dechlorinate dioxin-like PCBs.
This decreases a PCB’s binding with AhR and increases its water solubility, promoting expulsion from the body and therefore counteracting its toxicity. In other words, CYP is an important enzyme that determines whether or not PCBs are treated as toxic compounds by the body. To measure the metabolic action of CYP on chiral PCB, the researchers set up a CYP enzyme and PCB docking model. They used this to estimate the structure of PCB metabolites and the structure of the CYP that decides to metabolize each of the PCB atropisomers differently.
For the experiment, the group selected three types of chiral PCB, each with a different number of substituted chlorine atoms; CB45 (4 chlorine substitutions), CB91 (5 chlorine substitutions) and CB183 (7 chlorine substitutions). They separated the atropisomers for each type of chiral PCB using chromatography and let them react with a human CYP enzyme. It is believed that research on separating the atropisomers and letting them react has not been done before now.
The results revealed big differences in how each atropisomer is metabolized. Even though the two atropisomers in one PCB have the same physical and chemical composition, they are biologically different. The researchers found that one of the chiral PCB atropisomers was metabolized more than the other one, disrupting the 1:1 ratio.
In addition, it is thought that the amount of (aS)-CB183 atropisomer decreases because it is metabolized more than the other atropisomer, and this is supported by the reports of low accumulation of (aS)-CB183 in humans.
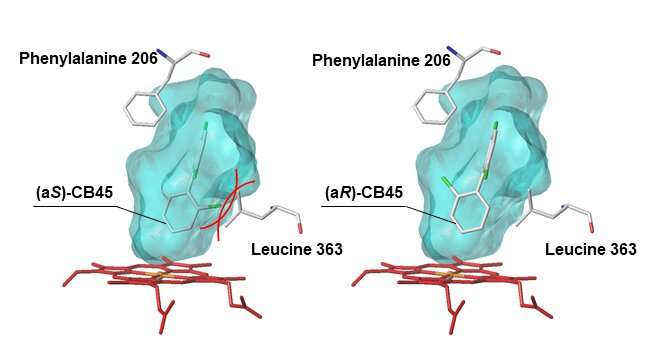
But why are these physically and chemically identical atropisomers metabolized differently by the CYP enzyme? To solve this mystery, the researchers used a computer model to investigate how easily each chiral PCB atropisomer binds to the chemical structure of CYP. They found that when an atropisomer fills up the substrate-binding cavity inside the CYP enzyme, CYP’s amino acids (that form the cavity) interfere with the binding between CYP and the atropisomer.
Therefore, the atropisomer that isn’t interfered with by CYP’s amino acids becomes easy to metabolize (atropisomer (aR)-CB45 in CB45, and (aS)-CB183 in CB183), resulting in alterations to the original 1:1 ratio of atropisomers found in chiral PCB.
The results of this research will be useful for making predictions about the atropisomers of chiral PCBs, which accumulate easily inside animals’ bodies. In other words, it will be possible to work out which atropisomer is reduced by the metabolic reaction with CYP enzymes and which atropisomer remains inside the body. A chiral PCB’s toxicity is activated by binding with RyR, though the ability to bind with RyR differs between the atropisomers. Therefore, this research will make it possible to estimate the toxicity of chiral PCBs.
More information: Hideyuki Inui et al, Differences in Enantioselective Hydroxylation of 2,2′,3,6-Tetrachlorobiphenyl (CB45) and 2,2′,3,4′,6-Pentachlorobiphenyl (CB91) by Human and Rat CYP2B Subfamilies, Environmental Science & Technology (2022). DOI: 10.1021/acs.est.2c01155
Terushi Ito et al, Enantioselective metabolism of chiral polychlorinated biphenyl 2,2′,3,4,4′,5′,6-Heptachlorobiphenyl (CB183) by human and rat CYP2B subfamilies, Chemosphere (2022). DOI: 10.1016/j.chemosphere.2022.136349
Journal information: Chemosphere , Environmental Science & Technology
Provided by Kobe University