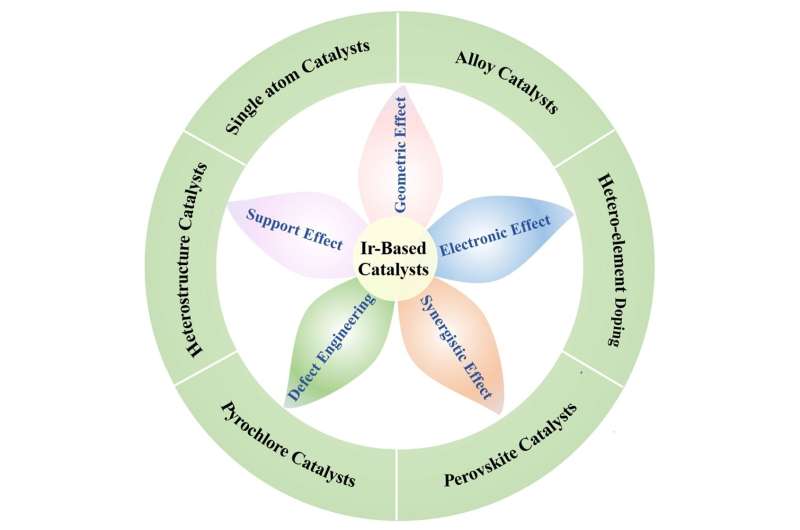
Hydrogen production powered by wind and solar energy is still too expensive if it is to play a role in the clean transition via energy storage and to help decarbonize hard-to-electrify sectors. Much effort in reducing its cost focuses on enhancing production efficiency by improving the performance of iridium-based catalysts that can speed up the oxygen-related part of the electrochemical reaction involved in splitting water into its component parts, hydrogen and oxygen.
A new review of the state of the field discusses its recent progress and challenges and identifies research gaps that need to be filled before such catalysts can achieve commercial viability.
The review paper was published in the journal Nano Research Energy.
Cleanly produced hydrogen is essential in the transition away from fossil fuels in order to avoid dangerous climate change, both as an energy carrier to be used on its own or as a component of a synthetic fuel for those sections of the economy such as long-haul shipping and aviation that are hard to electrify.
But such clean hydrogen production—which is performed via electrolysis, using electricity to split water into its component elements, hydrogen and oxygen—is extremely energy intensive. This energy intensity of electrolysis in turn makes clean production of hydrogen very expensive, and thus uncompetitive with fossil fuels.
If this were not enough of a challenge, using wind and solar energy as the source of clean electricity to power the electrolysis—a form of hydrogen production termed ‘green hydrogen’—places a significant burden on the electrolyzers because these energy sources are intermittent. The sun doesn’t always shine and the wind doesn’t always blow.
This means that sometimes there is little to no current and at other times, there can be a big spike of current, which places stress on the electrolyzers, again pushing up costs. However, proton exchange membrane water electrolyzers (PEMWE) are a very promising option here, as PEMWEs can operate at high current densities such as those posed by these spikes.
Electrolysis is a chemical reaction composed of two parts, or ‘half reactions.’ One is the hydrogen evolution reaction (HER), which generates the hydrogen, and the other is the oxygen evolution reaction (OER), which produces the oxygen. But it is actually the latter reaction that is most important with respect to the energy efficiency of the overall process and thus production of clean hydrogen.
And so to reduce the energy demands and thus the cost of clean production of hydrogen, a lot of research has focused on superior catalysts—chemicals that speed up a chemical reaction—for the OER part of the process and that pair well with PEMWEs.
However, the severe corrosion in the acidic environment of PEMWEs makes most non-precious metal-based catalysts—for example using cobalt, nickel, or iron—unstable. But iridium-based catalysts exhibit much better catalytic stability in these harsh acidic conditions.
A number of recent studies have reported significant advances in the development of iridium-based catalysts for green hydrogen production, including the use of new synthesis methods and the optimization of catalyst structures and compositions.
However, there are still several research challenges that need to be addressed to fully realize the potential of iridium-based catalysts for green hydrogen production. One major challenge is the high cost of iridium—and high costs are precisely what novel catalysts were intended to avoid.
“To overcome this, researchers are exploring new synthesis methods and alternative catalyst materials that can replace iridium or reduce the amount of iridium required,” said Chunyun Wang, of the School of Chemistry and Chemical Engineering at Yangzhou University and lead author of the review. “Some novel and effective options have emerged recently, such as iridium oxides, perovskites, pyrochlores, and single-atom catalysts.”
“And so we thought it was about time that we paused and assessed the state of play in iridium-based catalysts for green hydrogen production with a review paper,” added Alex Schechter, a chemist with Ariel University in Israel and co-author of the review paper. “The benefit of this is to pool information across many different teams of researchers and, crucially, identify research gaps.”
The review focuses in particular on how the catalysis operates (the catalytic mechanism), design of catalysts, and strategies for synthesis of catalysts. In particular, the analysis looks at different attributes of catalysts that affect their promotion of the catalysis process including geometric effects, electronic effects, synergistic effects, defect engineering and support effects, and how different research teams have dealt with each option to try to improve performance.
Geometric effects in essence describe the shape, structure and size of the catalyst molecule, including which of its crystal planes are exposed, and how atomic arrangements might be ordered or disordered. All of this significantly affects catalyst performance. Electronic effects refer to the structure of electrons associated with the relevant molecules.
Synergistic effects are those where two or more ingredients come together to produce a superior result than either one on its own. Defect engineering involves efforts to design the surface chemistry of catalysts via voids, dislocations, vacancies and so on—deliberately introducing imperfections—so as to increase the number of places where the chemical reaction can take place (active sites). And support effects come from metals that interact with and support the catalyst.
The reviewers concluded after surveying their field that the most successful strategy for improving the performance of iridium-based catalysts includes defect engineering, adjusting synergistic effects and altering geometric effects. The number of exposed active sites can be increased by constructing a porous structure and introducing supports for the catalyst that promote transfer of both mass and electrons. And enhanced metal-support interaction can increase the long-term stability of the catalysts.
Despite the considerable research success, the field still faces challenges. Many high-performance iridium-based catalysts have been developed, but most of them can only be synthesized on a small scale of just a few grams or even hundreds of milligrams in the laboratory. Complex preparation processes thus must be simplified.
In addition, lab conditions are a bit too ideal compared to actual catalytic systems, and so real-world conditions need to be part of any follow-up research. This includes looking at realistic electrolyzer temperature, current density, and product delivery, amongst other aspects, that will enable evaluation of performance catalysts in practical applications.
And beyond the catalysts themselves, other components need to be optimized as well, including the development of electrode plates with high corrosion resistance and low cost, proton exchange membranes with high proton transport capacity.
The reviewers stressed however that none of these challenges are deal-breakers for iridium-based catalysts for green hydrogen production. Instead these represent possible avenues for new research that may deliver the breakthroughs this process requires to achieve commercial viability.
More information: Chunyan Wang et al, Iridium-based catalysts for oxygen evolution reaction in acidic media: Mechanism, catalytic promotion effects and recent progress, Nano Research Energy (2023). DOI: 10.26599/NRE.2023.9120056
Provided by Tsinghua University Press