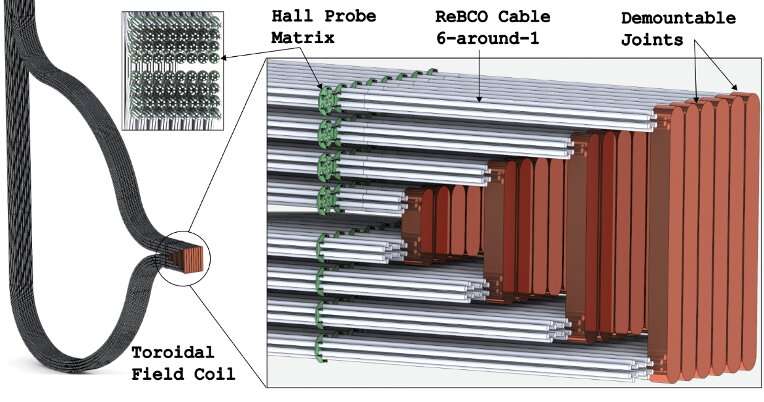
Researchers at Berkeley Lab’s Accelerator Technology & Applied Physics (ATAP) Division have developed a method for detecting and predicting the local loss of superconductivity in large-scale magnets that are capable of generating high magnetic fields. These high-field magnets are a core enabling technology for many areas of scientific research, medicine, and energy, where they are used in a range of applications, including in particle accelerators and colliders for high-energy and nuclear physics, diagnostic and therapeutic medical devices, and energy generation, transmission, and storage technologies.
High-field magnets also show promise as an enabling technology for magnetic confinement fusion reactors, which aim to replicate the processes that power the sun by fusing two hydrogen isotopes (deuterium and tritium) to produce a carbon-free source of energy. They are used to confine the plasma of deuterium and tritium so that fusion can occur.
To realize the full potential of these reactors “will require high-performance superconducting magnets capable of generating large magnetic fields safely and reliably under the demanding dynamic conditions found in fusion reactors,” says Reed Teyber, a Research Scientist at ATAP’s Superconducting Magnet Program who is developing diagnostic tools for monitoring the performance of both low- and high-temperature superconducting magnets. The work is published in the journal Scientific Reports.
Superconducting cables, however, can experience sudden and unpredictable losses in superconductivity—a phenomenon referred to as quenching—that can generate temperatures high enough to destroy the magnets, costing millions of dollars in damage.
“While for older, low-temperature superconducting magnets quenching is inherent, it must be avoided altogether in high-temperature superconducting magnets to ensure their reliable and safe operation,” explains Reed. “Detecting a quench and preventing it from damaging magnets is, therefore, a central focus for researchers looking to develop superconducting magnets for compact fusion reactors.”
Rare-earth barium copper oxide (ReBCO) is a promising material for the fabrication of superconducting magnets used in fusion reactors. ReBCO tape, which is used in the cables that carry the currents in superconducting magnets, has a high critical temperature (making it a so-called high-temperature superconductor), high critical field, and the potential to form demountable magnets—an important property that improves maintenance access, simplifies materials component testing, and allows for modularity to accommodate future reactor designs.
Superconducting magnets are familiar in such applications as colliders for high-energy physics, so methods exist for quench protection in low-temperature superconducting magnets like niobium-titanium or niobium-tin. These methods use voltage or temperature measurements to trigger energy extraction processes. However, Reed says quench detection in high-temperature superconducting magnets, like those that use ReBCO, needed for fusion reactors is far more challenging and calls for new approaches.
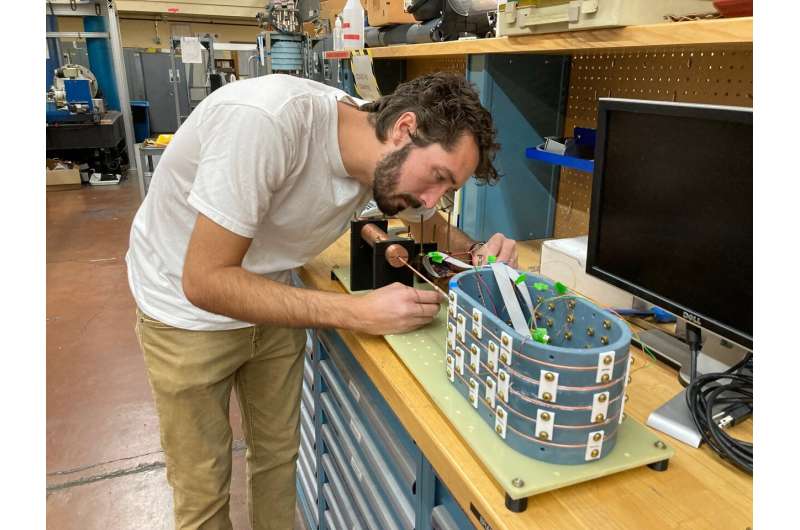
These extremely powerful magnets, capable of generating magnetic fields exceeding 20 tesla, are characterized by a much slower initial rate of quench development compared to accelerator magnets, making it difficult to detect quenches using voltage- or temperature-based techniques.
To address this issue, Reed is working with colleagues at ATAP to develop a method that employs an array of Hall probes—devices that are used to measure the magnetic fields created when a current-carrying conductor is placed in a magnetic field—to measure the magnetic fields produced around ReBCO CORC cables. These cables are composed of numerous “conductor-on-round-core” wires made from tapes of ReBCO to achieve the required current capacity.
Current distributions for the individual conductors recreated from these measurements provide insights into the detailed dynamics of magnet operation, allowing the extraction of parameters for a predictive model.
Although it shows promise as a powerful technique for quench detection and prevention, it currently has many limitations, notes Reed. “For example, it only works for specific magnet technologies with no inter-cable current sharing and cables of moderate length.”
However, he adds, it turns out that demountable toroidal field coils wound from ReBCO cables meet these requirements. Toroidal field coils are the leading superconducting magnet technology for generating the enormous magnetic fields required for containing the plasma to ensure fusion reactions can happen.
“We are now looking into how we can use our technique to solve the problem of quench detection in these coils.”
Reed says the work could be a “game-changer” not only for superconducting magnets used in nuclear fusion reactor experiments, but also for particle accelerators in high-energy physics, magnetic energy storage technologies, medicine, and various power devices such as electric motors, generators, and transmission lines.
“This innovative technique conceived by Reed,” said Paolo Ferracin, deputy program head of ATAP’s Superconducting Magnet Program, “has the potential to serve as a key element in solving the quench protection for high-temperature superconductor cables, a fundamental issue for the scientific community working on the next generation of superconducting magnets.”
More information: Reed Teyber et al, Current distribution monitoring enables quench and damage detection in superconducting fusion magnets, Scientific Reports (2022). DOI: 10.1038/s41598-022-26592-2
Journal information: Scientific Reports
Provided by Lawrence Berkeley National Laboratory