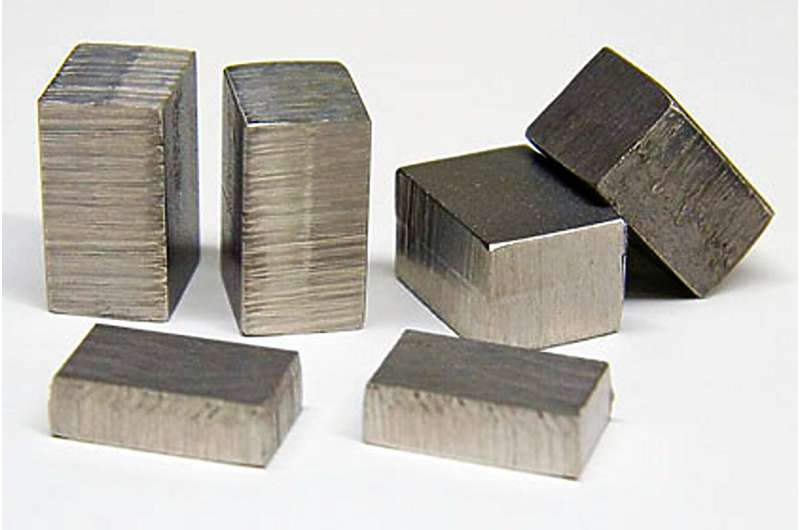
Nearly every material, whether it is solid, liquid, or gas, expands when its temperature goes up and contracts when its temperature goes down. This property, called thermal expansion, makes a hot air balloon float, and the phenomenon has been harnessed to create thermostats that automatically turn a home furnace on and off. Railroads, bridges, and buildings are designed with this property in mind, and they are given room to expand without buckling or breaking on a hot day.
Thermal expansion occurs because a material’s atoms vibrate more as its temperature increases. The more its atoms vibrate, the more they push away from their neighboring atoms. As the space between the atoms increases, the density of the material decreases and its overall size increases.
There are a few exceptions, but by and large, materials conform strictly to this principle. There is, however, a class of metal alloys called Invars (think “invariable”), that stubbornly refuse to change in size and density over a large range of temperatures.
“It’s almost unheard of to find metals that don’t expand,” says Stefan Lohaus, a graduate student in materials science and lead author of the new paper. “But in 1895, a physicist discovered by accident that if you combine iron and nickel, each of which has positive thermal expansion, in a certain proportion, you get this material with very unusual behavior.”
That anomalous behavior makes these alloys useful in applications where extreme precision is required, such as in the manufacture of parts for clocks, telescopes, and other fine instruments. Until now, no one knew why Invars behave this way. In a new paper titled “Thermodynamic explanation of the Invar effect,” published in Nature Physics, researchers from the lab of Brent Fultz, the Barbara and Stanley R. Rawn, Jr., Professor of Materials Science and Applied Physics, say they have figured out the secret to at least one Invar’s steadiness.
For over 150 years, scientists have known that thermal expansion is related to entropy, a central concept in thermodynamics. Entropy is a measure of the disorder, such as positions of atoms, in a system. As temperature increases, so does the entropy of a system. This is universally true, so an Invar’s unusual behavior must be explained through something counteracting that expansion.
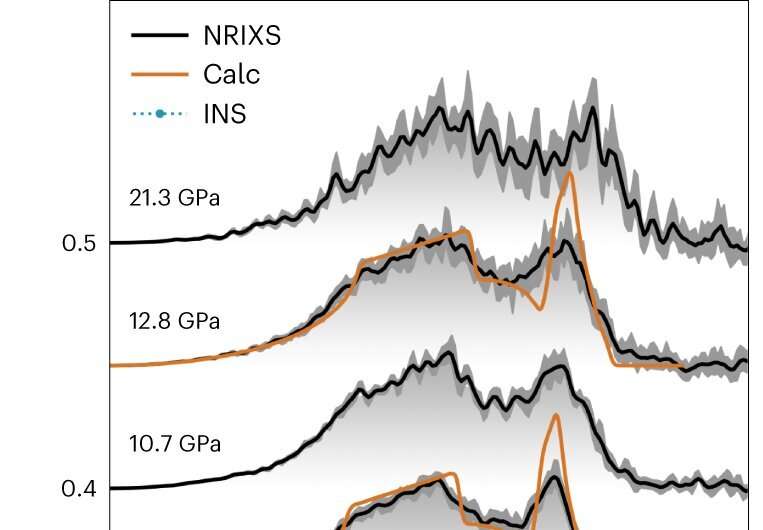
Lohaus says it had been long suspected that this behavior was somehow related to magnetism because only certain alloys that are ferromagnetic (capable of being magnetized) behave as invars.
“We decided to look at that because we have this very neat experimental setup that can measure both magnetism and atomic vibrations,” Lohaus says. “It was a perfect system for this.”
Since the magnetic properties of a material are the result of its electrons’ so-called spin state— a quantum measure of angular momentum that can be either “up” or “down”—any magnetic effect counteracting the material’s expected expansion must be due to the activity of its electrons.
The relationship between entropy, thermal expansion, and pressure, known as the “Maxwell relations” is often presented as a textbook curiosity, but the Caltech group found a way to use it to independently measure the thermal expansion caused by magnetism and by atom vibrations. The experiments were done at the Advanced Photon Source, a source of synchrotron X-rays at the Argonne National Laboratory in Illinois, by measuring the vibrational spectra and magnetism of small samples of Invar at pressures within a diamond anvil cell.
The measurements showed a delicate cancelation of the thermal expansion from atom vibrations and from magnetism. Both changed with temperature and pressure, but in a way that maintained their balance. Using a newly developed accurate theoretical approach, collaborators on this work showed how this balance was helped by interactions between vibrations and magnetism, such as where the frequencies of atom vibrations are altered by magnetism. Such coupling between vibrations and magnetism could be useful for understanding thermal expansion in other magnetic materials, as well for developing materials for magnetic refrigeration.
The experimental setup consisted of a diamond anvil cell, which is essentially two precisely ground diamond tips between which samples of materials can be tightly squeezed. In this case, a small piece of Invar alloy was squeezed at a pressure of 200,000 atmospheres. The researchers passed a powerful beam of X-rays through the alloy, and during that process the X-rays interacted with the vibrations (phonons) of its atoms. That interaction changed the amount of energy carried by the X-rays, allowing the researchers to measure how much the atoms were vibrating.
They also placed sensors around the diamond anvil cell that can detect interference patterns created by the spin state of the electrons belonging to the sample’s atoms.
The team used their experimental setup to observe both the atomic vibrations of an Invar sample and the spin state of its electrons as they increased the sample’s temperature. At cooler temperatures, more of the Invar’s electrons shared the same spin state, causing them to move farther apart and push their parent atoms farther apart as well.
As the temperature of the Invar rose, the spin state of some of those electrons increasingly flipped. As a result, the electrons became more comfortable cozying up to their neighboring electrons. Typically, this would cause the Invar to contract as it warmed up. But here, the Invar’s atoms were also vibrating more and taking up more room. The contraction due to changing spin states and the atomic vibration expansion counteracted each other, and the Invar stayed the same size.
“This is exciting because this has been a problem in science for over a hundred years or so,” Lohaus says. “There are literally thousands of publications trying to show how magnetism causes contraction, but there was no holistic explanation of the Invar effect.”
Co-authors are graduate students in materials science Pedro Guzman and Camille M. Bernal-Choban, visitor in applied physics and materials science Claire N. Saunders, Guoyin Shen of the Argonne National Laboratory, Olle Hellman of the Weizmann Institute of Science, David Broido and Matthew Heine of Boston College, and Fultz.
More information: S. H. Lohaus et al, A thermodynamic explanation of the Invar effect, Nature Physics (2023). DOI: 10.1038/s41567-023-02142-z
Journal information: Nature Physics
Provided by California Institute of Technology