by Anne Trafton, Massachusetts Institute of Technology
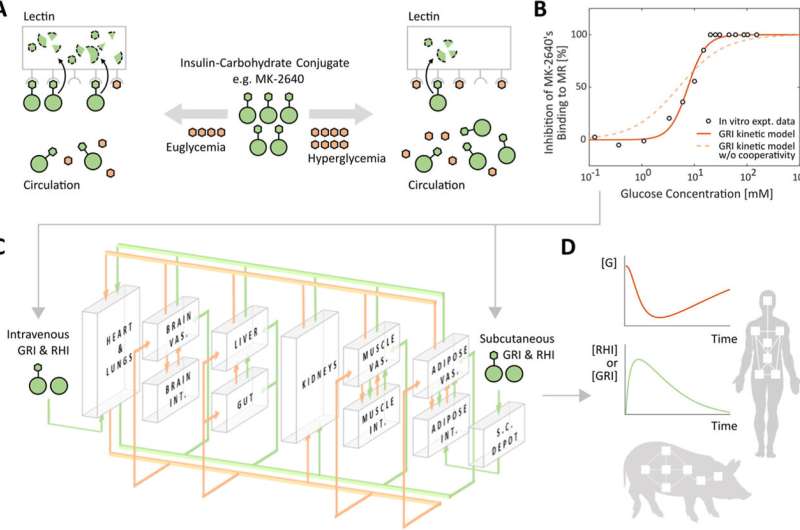
For diabetes patients who must give themselves frequent insulin injections, the risk of low blood sugar can be life-threatening. A potential solution is a type of engineered insulin that circulates in the body and springs into action only when needed. Researchers working on this type of “glucose-responsive insulin” (GRI) hope that it could be injected less often and help the body maintain normal blood sugar levels for longer periods of time.
To help in the efforts to develop this kind of insulin, MIT engineers have created a computational model that predicts how the human body will respond to different versions of GRIs. Their model is unique in that it can also compare the human response to those of lab animals used for preclinical testing of GRIs.
In a new study, the MIT team used the model to analyze the results of a recent GRI clinical trial that was discontinued because the drug showed little effect in humans. Their analysis found that the drug, which had worked well in animal studies, acted differently in the human body because of differences in the behavior of a sugar receptor that helps to control the drug’s action.
Using this model, researchers could design novel GRIs and obtain better predictions of whether a particular GRI would work in humans before launching a costly clinical trial.
“This model can help with the design process and also help to predict human performance, which I think is going to de-risk the investment of taking these types of drugs to clinical trials,” says Michael Strano, the Carbon P. Dubbs Professor of Chemical Engineering at MIT and the senior author of the new study, which appears in ACS Pharmacology and Translation.
Jingfan Yang Ph.D. is the lead author of the paper, working with current graduate student Sungyun Yang.
Modeling glucose response
In recent years, many drug companies have been working on glucose-responsive insulins, which are activated in response to high blood sugar levels. In 2016, the pharmaceutical company Merck ran a phase I clinical trial of a GRI called MK-2640, which had shown promising results in preclinical studies performed in animals.
“This drug, if it were to show efficacy in clinical trials and then go to the market, would have helped diabetics because it’s a therapeutic that can activate as their blood sugar rises,” Strano says. “This gives them a better degree of control and can provide protection against hypoglycemia or dangerously low blood sugar.”
MK-2640 was designed with a novel glucose-response mechanism known as competitive clearance. The insulin is engineered so that it binds to cell receptors that normally bind to a sugar called mannose. When blood sugar levels are low, GRI molecules bind to these receptors and are cleared from the body. However, when blood sugar rises, the GRI is blocked from binding to the receptors and stays in the bloodstream, where it helps to reduce sugar levels.
“This is a great way of building pancreatic function into the drug. It’s been a longstanding goal of the diabetes community to make a drug that operates in this way,” Strano says. “The drug performed very well in animals, but when it was tried in humans, it showed a really lackluster effect.”
Around the same time, Strano’s lab was developing a way to computationally model the glucoregulatory system of humans and other animals. The model consists of a set of equations that describes how glucose and insulin behave in different compartments of the human body, such as blood vessels, muscle, and fatty tissue. This allows them to predict blood glucose levels in organs including the liver, stomach, and brain, for a variety of species, including humans.
“This is a very detailed model, and it has parameters that have been tuned with extensive clinical data and animal data, so it’s able to faithfully recreate experiments that researchers do on both humans and animals,” Strano says.
For example, the model can be used to predict how blood sugar levels will change after a meal, or what will happen if glucose is infused into the body, based on how much insulin is available. His lab has previously used this model to predict the behavior of a different type of glucose-responsive insulin, which is coated with molecules called PBA that bind to glucose and activate insulin.
Designing better drugs
After the Merck trial ended, Strano and his students decided to see if their computational model could generate any insight into why the drug did not perform as expected. They applied it to all of the data available on the glucoregulatory system of Yucatan minipigs, the animal model used for some of the Merck preclinical studies.
Using the computational model, the researchers found that interspecies differences in the clearance capacity of the mannose receptor accounted for the weak performance of the GRI in human trials. Because of that difference, GRI levels did not change significantly in humans compared to animals when glucose levels changed.
“It’s really a failure of animal models to capture an important element of the mechanism in humans,” Strano says.
The model also showed that if the human version of the mannose receptor were tuned to perform similarly to that of the minipigs, the drug would likely have performed much better in clinical trials.
The researchers have made their model open source so that others can use it to explore potential GRIs that might work better, and to evaluate whether drugs that have shown promise in animal studies would also work well in humans.
Strano’s lab is also working on such drugs, in collaboration with Michael Weiss, chair of the Department of Biochemistry and Molecular Biology at Indiana University, who is also an author of the ACS Pharmacology and Translation study.
Strano’s lab is also working on another version of the model that would incorporate the effects of glucagon, a hormone that increases blood sugar and can prevent life-threatening hypoglycemia. Researchers have theorized that treating diabetic patients with a combination of insulin and glucagon could offer better control of blood sugar levels than insulin alone.
The general approach of designing drugs that respond to conditions inside the body could also be beneficial for treating a wide variety of other diseases, Strano says.
“This could lead to a new generation of drugs that don’t just passively circulate within the body and wait to operate, but are tuned to reach a certain therapeutic endpoint and regulate their potency accordingly,” he says.
More information: Jing Fan Yang et al, In Silico Investigation of the Clinical Translatability of Competitive Clearance Glucose-Responsive Insulins, ACS Pharmacology & Translational Science (2023). DOI: 10.1021/acsptsci.3c00095
Provided by Massachusetts Institute of Technology