by Nicola Nosengo, National Centre of Competence in Research (NCCR) MARVEL
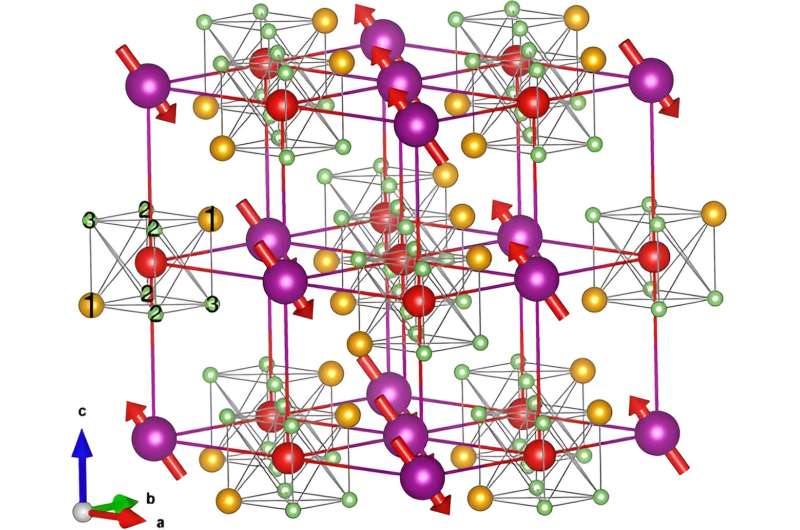
Muon spectroscopy is an important experimental technique that scientists use to study the magnetic properties of materials. It is based on “implanting” a spin-polarized muon in the crystal and measuring how its behavior is affected by the surroundings.
The technique relies on the idea that the muon will occupy a well-identified site that is mainly determined by electrostatic forces, and that can be found by calculating the material’s electronic structure.
But a new study led by scientists in Italy, Switzerland, UK and Germany has found that, at least for some materials, that is not the end of the story: the muon site can change due to a well-known but previously neglected effect, magnetostriction.
Pietro Bonfà from the University of Parma, lead author of the study published in Physical Review Letters, explains that his group and their colleagues at the University of Oxford (UK) have been using density-functional theory (DFT) simulations for at least a decade to find muon sites.
“We started with tricky cases, such as europium oxide and manganese oxide, and in both cases, we could not find a reasonable way to reconcile DFT predictions and the experiments,” he says.
“We then tested simpler systems and we had many successful predictions, but those two cases were really bothering us. These compounds should be easy and instead turned out to be super complicated and we did not understand what was happening. Manganese oxide is a textbook case of an antiferromagnetic system, and we could not explain muon spectroscopy results for it, which was a bit embarrassing.”
The problem, he explains, was the contradiction between the expectation to find the muon in a high symmetry position, and its well-known tendency to make bonds with oxygen atoms. The antiferromagnetic order of the material reduces the symmetry, and the position close to the oxygen atoms becomes incompatible with experiments.
Bonfà suspected that the explanation could be linked to the material undergoing a magnetic phase transition and started trying to reproduce the phenomenon in simulations of manganese oxide.
“Because it is a complicated system, you must add some corrections to DFT, such as the Hubbard U parameter,” he said. “But we were choosing its value empirically, and when you do that, you have a lot of uncertainty, and the results can change dramatically depending on the value you choose.”
Still, Bonfà’s initial simulations suggested that the muon positions could be driven by magnetostriction, a phenomenon that causes a material to change its shape and dimensions during magnetization. To prove it beyond doubt, he teamed up with the MARVEL laboratories at EPFL and PSI of Nicola Marzari and Giovanni Pizzi.
“We used a state-of-the-art method called DFT+U+V, which was very important to make simulations more accurate,” explains Iurii Timrov, a scientist in the Laboratory for Materials Simulations at PSI and co-author of the study.
This method can be used with onsite U and intersite V Hubbard parameters that are computed from first principles instead of being chosen empirically, thanks to the use of density-functional perturbation theory for DFT+U+V that was developed within MARVEL and implemented in the Quantum ESPRESSO package.
“Although we had already figured out that magnetostriction was at play, having the correct information on the building blocks of the simulation was very important, and that came from Iurii’s work,” adds Bonfà.
In the end, the solution of the puzzle was relatively simple: magnetostriction, which is the interplay between magnetic and elastic degrees of freedom in the material, causes a magnetic phase transition in MnO at 118K, at which the muon site switches. Above that temperature, the muon becomes delocalized around a network of equivalent sites—which explains the unusual behavior observed in experiments at high temperatures.
The scientists expect that the same may be true also for many other rocksalt-structured magnetic oxides.
In the future, Timrov explains, the group wants to keep studying the same material also including temperature effects, using another advanced technique developed in MARVEL and called stochastic self-consistent harmonic approximation.
In addition, and in collaboration with Giovanni Pizzi’s group at the Paul Scherrer Institute, this approach will be made available to the community through the AiiDAlab interface, so that all experimentalists can use it for their own studies.
More information: Pietro Bonfà et al, Magnetostriction-Driven Muon Localization in an Antiferromagnetic Oxide, Physical Review Letters (2024). DOI: 10.1103/PhysRevLett.132.046701
Journal information: Physical Review Letters
Provided by National Centre of Competence in Research (NCCR) MARVEL
