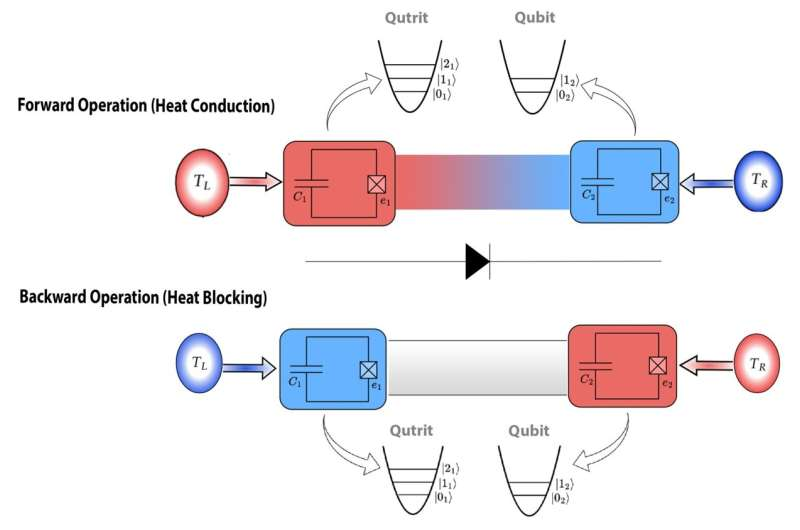
Heat management at the nanoscale has long been a cornerstone of advanced technological applications, ranging from high-performance electronics to quantum computing. Addressing this critical challenge, we have been deeply intrigued by the emerging field of thermotronics, which focuses on manipulating heat flux in ways analogous to how electronics control electric energy. Among its most promising advancements are quantum thermal diodes, which enable directional heat control, and quantum thermal transistors, which regulate heat flow with precision.
Thermal diodes, much like their electrical counterparts, provide unidirectional heat transfer, allowing heat to flow in one direction while blocking it in the reverse. We find this capability revolutionary for heat management, as it has the potential to transform numerous fields.
For instance, thermal diodes can significantly improve the cooling of high-performance electronics, where heat dissipation is a major bottleneck. They could also enable more efficient energy harvesting by converting waste heat into usable energy, contributing to sustainability efforts.
Additionally, they offer applications such as dynamically managing building temperatures, enhancing the performance of thermoelectric generators, or even improving spacecraft thermal systems, where precisely controlled heat flow is critical.
In our research, we have noticed that most quantum thermal device models to date have relied on simple quantum systems with two stable energy levels, such as qubits. However, we see significant potential to go beyond these limitations.
At the Advanced Computing and Simulation Laboratory (AχL), Monash University, Australia, we have been exploring higher-dimensional quantum systems that expand the capabilities of these devices. By integrating qubit-qutrit architectures, we have demonstrated directional heat flow with improved efficiency and scalability.
This breakthrough, published in APL Quantum, lays the groundwork for practical, high-performance thermotronic systems that could address challenges ranging from overheating in modern technology to advancing sustainable energy solutions. These advancements represent a critical step forward, promising to redefine heat management and energy efficiency in the quantum era.
Harnessing quantum asymmetry to regulate unidirectional heat flow
The quantum thermal diode, based on the interaction between a qutrit (a quantum system with three stable energy levels) and a qubit (a system with two stable energy levels), introduces a novel approach to unidirectional heat transfer.
This system leverages the inherent properties of quantum mechanics to create an asymmetric energy landscape that naturally favors heat flow in one direction, depending on the temperature gradient. This directional behavior is analogous to the way an electronic diode facilitates unidirectional current flow based on the potential difference across its terminals.
The key to this thermal diode lies in how the energy levels of the qubit and qutrit align and interact. By carefully configuring the combined energy levels, we can facilitate heat transfer along the desired temperature gradient while effectively blocking it in the opposite direction. This directional control is achieved through precise quantum interactions, which utilize specific shared energy levels between the qubit and qutrit to establish the necessary conditions for asymmetry in heat flow.
What makes this system particularly groundbreaking is its ability to operate as a nearly perfect thermal diode across a broad temperature range. Unlike classical thermal systems, the quantum nature of this device allows for precise tuning of its properties, including the spacing of energy levels and the coupling strengths between the qubit and qutrit. This tunability enables unprecedented control over the heat transfer process, making the device highly adaptable to various applications.
Whether improving the heat management of nanoscale devices or developing next-generation thermotronic systems, we believe this architecture represents a major step forward in thermal management technologies. By combining a qutrit and a qubit into a single system, this design not only achieves directional heat flow but also enhances efficiency, offering a practical and scalable solution for advanced thermotronics.
Shaping future technologies: The transformative potential of quantum thermal diodes
The development of a quantum thermal diode is a transformative breakthrough with significant implications for quantum thermodynamics and nanoscale engineering. By enabling precise control of heat flow at the quantum level, this innovation addresses challenges that traditional cooling methods cannot solve, particularly in quantum circuits and advanced nanoscale devices.
For example, quantum thermal diodes can regulate heat dissipation in quantum processors, ensuring stable and optimal performance where even slight overheating could lead to disruptions. Additionally, they open up new opportunities for energy harvesting by capturing waste heat generated in quantum systems and converting it into usable energy. This capability has the potential to drive sustainable energy solutions across numerous applications.
Beyond energy efficiency, we believe quantum thermal diodes could pave the way for thermal logic devices—thermal analogs to electronic diodes—allowing computation to be performed using heat flow rather than electric current. Such a development would represent an entirely new paradigm in computation, with applications in fields requiring unique architectures for energy and heat management.
Furthermore, these devices hold significant promise in specialized areas, such as biomedical technologies, where precise thermal regulation is critical for maintaining the performance of sensitive quantum sensors. They could also prove vital in space exploration, where managing the temperature of delicate quantum instruments in extreme environments is essential.
By improving the efficiency of heat dissipation and enabling directional control, quantum thermal diodes not only enhance the functionality of nanoscale devices but also set the stage for the next generation of technologies.
With the potential to develop quantum thermal transistors and other advanced thermotronic devices, we believe this innovation has the power to redefine how we approach thermal management and energy utilization in a quantum-driven world. From nanoscale engineering to space exploration, the transformative potential of quantum thermal diodes promises to shape the technologies of tomorrow.
This story is part of Science X Dialog, where researchers can report findings from their published research articles. Visit this page for information about Science X Dialog and how to participate.
More information: Anuradhi Rajapaksha et al, Enhanced thermal rectification in coupled qutrit–qubit quantum thermal diode, APL Quantum (2024). DOI: 10.1063/5.0237842
Bios:
Anuradhi Rajapaksha earned her B.Sc. in electrical and electronic engineering (with first-class honors) from University of Peradeniya, Sri Lanka in 2021. Currently she is a PhD candidate and a member of the Advanced Computing and Simulations Laboratory at the Department of Electrical and Computer Systems Engineering, Monash University, Australia under the supervision of Prof. Malin Premaratne.
Sarath D. Gunapala received a Ph.D. degree in physics from the University of Pittsburgh, Pittsburgh, PA, USA, in 1986. In 1992, he joined NASA’s Jet Propulsion Laboratory, California Institute of Technology, Pasadena, CA, USA, where he is currently the Director of the Center for Infrared Photodetectors. He is also a Senior Research Scientist and a Principal Member of the Engineering Staff with the NASA Jet Propulsion Laboratory.
Malin Premaratne earned several degrees from the University of Melbourne, including a B.Sc. in mathematics, a B.E. in electrical and electronics engineering (with first-class honors), and a PhD in 1995, 1995, and 1998, respectively. Currently, he is a full professor at Monash University Clayton, Australia. His expertise centers on quantum device theory, simulation, and design, utilizing the principles of quantum electrodynamics.
Journal information: APL Quantum