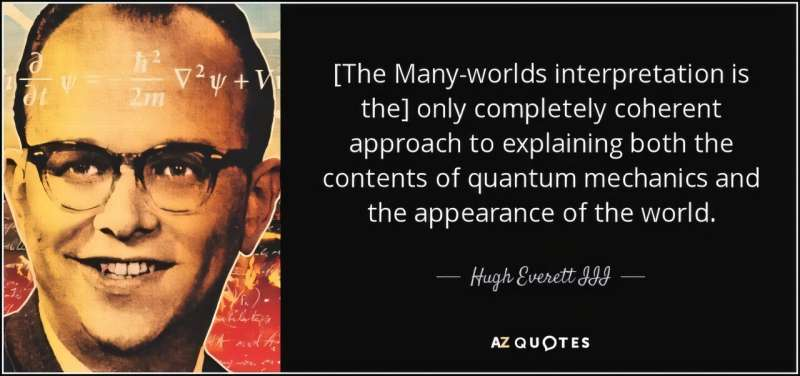
Students learning quantum mechanics are taught the Schrodinger equation and how to solve it to obtain a wave function. But a crucial step is skipped because it has puzzled scientists since the earliest days—how does the real, classical world emerge from, often, a large number of solutions for the wave functions?
Each of these wave functions has its individual shape and associated energy level, but how does the wave function “collapse” into what we see as the classical world—atoms, cats and the pool noodles floating in the tepid swimming pool of a seedy hotel in Las Vegas hosting a convention of hungover businessmen trying to sell the world a better mousetrap?
At a high level, this is handled by the “Born rule”—the postulate that the probability density for finding an object at a particular location is proportional to the square of the wave function at that position.
Erwin Schrödinger invented his famous feline as a way to amplify the consequences of the collapsing wave function—a simple event, such as a quantum event of the radioactive decay of an atomic nucleus, somehow translates into the macroscopic cat in the box being, either alive or dead. (This mysterious transition, perhaps theoretical only, is called the Heisenberg Cut.)
Traditional quantum mechanics says that at any time the cat becomes either alive or dead when the box is opened and the cat state is “measured.” Before that, the cat is, in a sense, both alive and dead—it exists in a quantum superposition of each state. It is only when the box is opened and its inside is viewed does the wave function of the cat collapse into a definite state of being either alive or dead.
In recent years, physicists have been looking at this process more deeply to understand what’s happening. Modifying the Schrödinger equation has had only limited success.
Other ideas than the Copenhagen interpretation described above, such as De Broglie-Bohm pilot wave theory and the many-worlds interpretation of quantum mechanics, are receiving more attention.
Now a team of quantum theorists from Spain have used numerical simulations to show that, on large scales, features of the classical world can emerge from a wide class of quantum systems. Their work is published in the journal Physical Review X.
“Quantum physics is at odds with our classical experience as far as the behavior of single electrons, atoms or photons is concerned,” lead author Philipp Strasberg of the Autonomous University of Barcelona told Phys.org.
“However, if one zooms out, and considers coarse quantities that we humans can perceive (for example, the temperature of our morning coffee or the position of a stone), our results indicate that quantum interference effects, which are responsible for weird quantum behavior, vanish.”
Their finding suggests that the classical world we see can emerge from the many-worlds picture of quantum mechanics, where many universes exist at the same point in spacetime and where almost a potentially huge number of worlds branch off from ours every time a measurement is made.
As a rough analogy, imagine a shower bag filled with water. Poke holes in the bag and water—which inside the bag is a large collection of frequently colliding molecules moving in random directions—will stream out in mostly smooth flows. This is akin to how the complicated jumble of a quantum system nonetheless appears in the classical world as something we recognize and feel familiar with.
But a technical problem remained with the many-worlds portrait: how do we reconcile the many-universes with the classical experience we have within our one universe? After all, we never see cats in a superposition of alive and dead. A priori, how can we speak of other universes or worlds or branches in any meaningful sense?
In their paper, Strasberg and co-authors write “Speaking of different worlds or histories becomes meaningful if we can reason about their past, present, and future in classical terms.”
The co-authors attempted to solve this problem in a new way. While previous work has brought in the idea of quantum decoherence—where the objects we see arise out of the many superpositions of a quantum system when it interacts with their environment. But this approach has a fine-tuning problem—it only works for specific types of interactions and types of initial wave functions.
By contrast, the group showed that a stable, self-consistent set of features emerges from the range of many possible evolutions of a wave function (with many energy levels) at observable, non-microscopic scales. This solution does not have a fine-tuning problem, works for a wide choice of initial conditions and the details of the interactions between energy levels.
“In particular,” Strasberg told Phys.org, “we provide clear evidence that this vanishing [of quantum interference effects] happens extremely fast—to be precise: exponentially fast—with growing system size. That is, even a few atoms or photons can behave classically. Furthermore, it is a ubiquitous and generic phenomenon that does not require any fine tuning: the emergence of a classical world is inevitable.”
The group numerically simulated quantum evolution for up to five time-steps and up to 50,000 energy levels for nontrivial quantum systems. Though that evolution is still small compared to what will be needed to simulate everyday classical phenomena, it’s much larger than any previous work.
They considered a broad range of choices of the initial wave function and of coupling strengths and found approximately the same large-scale structure of stable branches exist—the emergence of a stable and slowly evolving macroscopic structure.
“Remarkably, we also explicitly demonstrate that interesting classical worlds can emerge from a quantum system that is overall in thermodynamic equilibrium. Even though it is very unlikely that this is the case in our universe, it nevertheless demonstrates that order, structure and an arrow of time can emerge on single branches of a quantum Multiverse, which overall looks chaotic, unstructured and time-symmetric.”
Relating their work to statistical mechanics, where macroscopic features like temperature and pressure emerge from a melange of randomly moving particles, the group found that some branches lead to worlds where entropy increases and others to worlds where entropy decreases. Such worlds would have opposite entropic arrows of time.
More information: Philipp Strasberg et al, First Principles Numerical Demonstration of Emergent Decoherent Histories, Physical Review X (2024). DOI: 10.1103/PhysRevX.14.041027
Journal information: Physical Review X
© 2024 Science X Network