by Zhang Nannan, Chinese Academy of Sciences
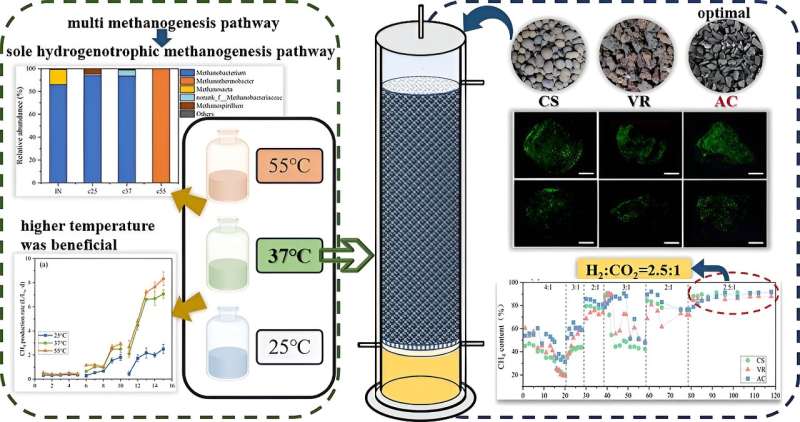
Biological hydrogen-methane conversion refers to the production of methane through the action of microorganisms using hydrogen generated by electrolysis of water with residual power and carbon dioxide present in biogas. This approach promises to overcome the limitations of hydrogen storage, lowering the financial burden of biogas upgrading, and enabling carbon-negative utilization of CO2 in biogas.
Previously, researchers from the Qingdao Institute of Bioenergy and Bioprocess Technology of the Chinese Academy of Sciences have domesticated and obtained microorganisms with high hydrogen-methane conversion efficiencies. They have also developed two production processes for in-situ and ex-situ biological hydrogen-methane conversion. However, the main factor limiting the efficiency of hydrogen-methane conversion remains the low gas-liquid mass transfer rate of hydrogen.
To address the limitations of low hydrogen mass transfer rates in the hydrogen-methane conversion process, the researchers developed a biotrickling filter (BTF), which facilitates microorganisms growth by using packing material with a rough internal surface. It ensures full contact between the gas and liquid phases, thereby increasing the efficiency of hydrogen utilization.
The study ia published in Chemical Engineering Journal.
In this study, the researchers started by exploring the effects of temperatures (25°C, 37°C, and 55°C) on the hydrogen-methane conversion pathway to determine the optimal temperature for the biotrickling filters. During the operation of the biotrickling filter, the effects of the packing materials (ceramite, volcanic stone, activated carbon) and the optimal ratio of the input gas (H2/CO2, v/v) on the conversion process were evaluated.
According to the researchers, the selected packing materials were environmentally friendly, and their large specific surface area and porosity facilitated the growth and attachment of microorganisms. This ensures sufficient contact between the microorganisms and the gas phase, which greatly enhances gas-liquid mass transfer.
The results showed that higher temperature is conducive to hydrogen-methane conversion. At 25°C, the hydrogen-methane conversion efficiency was low (2.5 L/Lw·d), and most of the hydrogen and carbon dioxide were used to produce acetate.
At 55°C, although the reaction process was initially unstable, it eventually reached stability and obtained a hydrogen-methane conversion efficiency of 8.3 L/Lw·d. In contrast, the conversion efficiency was still substantial at 37°C, achieving 7.1 L/Lw·d. Notably, there was no significant difference in the overall methanogenesis process between 37°C and 55°C.
In addition, the optimal input gas (H2/CO2) ratio was determined in the BTF experiment, achieving the most satisfactory ratio at 2.5:1 (H2/CO2, v/v), which was lower than previously reported values, but higher carbon dioxide removal efficiency was achieved.
The biofilms adhering to the three packing materials all achieved effective hydrogen-methane conversion efficiency at the ratio of 2.5:1, with the BTF using activated carbon as the packing material achieved the highest and the most stable conversion efficiency (91.9%).
The relative fluorescence intensity measurement confirmed that activated carbon had superior microbial immobilization. This study provides a promising approach for the application of BTFs in biogas hydrogen-methane conversion.