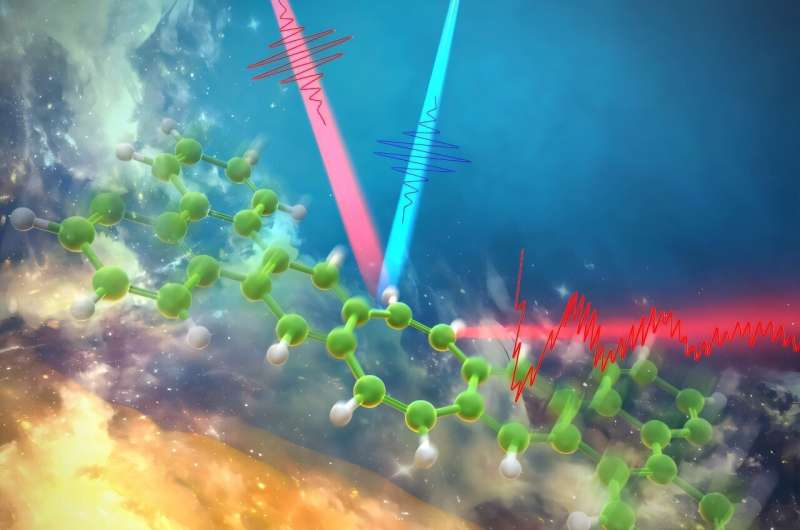
Scientists have achieved the first real-time visualization of how excited-state aromaticity emerges within just hundreds of femtoseconds and then triggers a molecule to change from bent to planar structure in a few picoseconds.
By combining ultrafast electronic and vibrational spectroscopies, the team captured these fleeting structural changes at the molecular level and showed that aromaticity appears before—and then drives—the structural planarization. Their findings lay the groundwork for designing more efficient photoactive materials, such as sensors and light-driven molecular switches, by leveraging the power of aromaticity in excited states.
The study is published in the Journal of the American Chemical Society. The research was led by Hikaru Kuramochi, Associate Professor at the Institute for Molecular Science/SOKENDAI.
Aromaticity is a foundational concept in chemistry describing the enhanced stability of cyclic molecules whose electrons are delocalized. Although most discussions have focused on molecules in their ground state, the concept of excited-state aromaticity has recently been extensively utilized in predicting the structural change and designing the chemical reactivities induced by photoexcitation.
While the dynamic properties of excited-state aromaticity have been studied intensively in the past, these have primarily focused on molecules in a near-equilibrium state, leaving the precise timing and interplay between excited-state aromaticity and structural changes poorly understood. Directly visualizing these ultrafast motions is crucial for designing photoactive materials, such as sensors, adhesives, and switches.
The team used a combination of femtosecond transient absorption and time-resolved impulsive stimulated Raman spectroscopy (TR-ISRS)—an advanced time-domain Raman technique that covers vibrational frequencies from terahertz to 3000 cm⁻¹ with femtosecond temporal resolution—to capture ultrafast snapshots of a newly synthesized cyclooctatetraene (COT)-based flapping molecule called TP-FLAP.
By exciting TP-FLAP with a femtosecond laser pulse, then probing its evolving vibrational signals, they could see exactly when and how the molecule’s central COT ring planarized. Isotope labeling with ¹³C at the central ring allowed the researchers to confirm which specific vibrational mode accompanied the bent-to-planar transition.
Initial measurements revealed a sub-picosecond (≈590 fs) electronic relaxation that imparts aromatic character to the bent molecule’s excited state. The molecule then undergoes planarization in a few picoseconds as indicated by a frequency shift in the ring’s carbon-carbon stretching vibration.
With the help of the isotope labeling (¹³C), a telltale shift in the key C=C stretching frequency was unambiguously shown, confirming that the ring’s planarization drives the observed vibrational changes. Calculations of aromaticity indices (e.g., nucleus-independent chemical shifts, NICS) further support that the system is already aromatic in the bent excited state and becomes even more aromatic as it undergoes planarization.
This study provides the first direct observation of nonequilibrium structural changes governed by excited-state aromaticity. It conclusively shows that aromaticity can emerge within hundreds of femtoseconds, preceding—and then facilitating—the picosecond-scale flattening of the molecule.
Beyond deepening our understanding of fundamental light-driven processes, these insights help guide the rational design of photoactive materials, including molecular sensors, tunable fluorescence probes, and photoresponsive adhesives. The TR-ISRS method’s ability to track vibrational modes in real time offers a new avenue for exploring other systems featuring excited-state (anti)aromaticity and complex conformational changes.
More information: Yusuke Yoneda et al, Excited-State Aromatization Drives Nonequilibrium Planarization Dynamics, Journal of the American Chemical Society (2025). DOI: 10.1021/jacs.4c18623
Journal information: Journal of the American Chemical Society
Provided by National Institutes of Natural Sciences