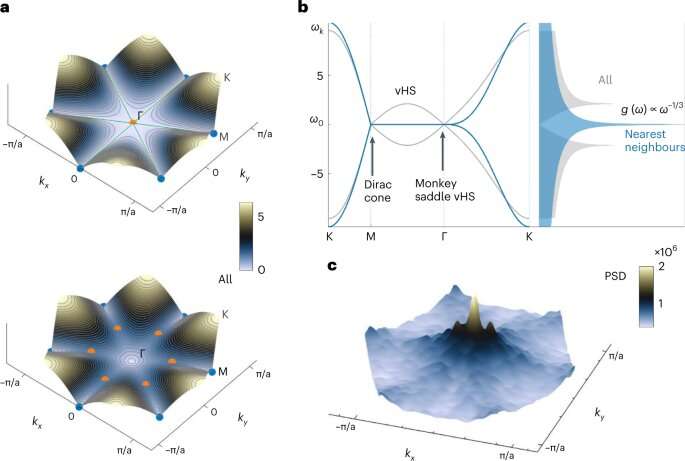
Starting with the emergence of quantum mechanics, the world of physics has been divided between classical and quantum physics. Classical physics deals with the motions of objects we typically see every day in the macroscopic world, while quantum physics explains the exotic behaviors of elementary particles in the microscopic world.
Many solids or liquids are composed of particles interacting with one another at close distances, which sometimes results in the rise of “quasiparticles.” Quasiparticles are long-lived excitations that behave effectively as weakly interacting particles. The idea of quasiparticles was introduced by the Soviet physicist Lev Landau in 1941, and ever since has been highly fruitful in quantum matter research. Some examples of quasiparticles include Bogoliubov quasiparticles (i.e. “broken Cooper pairs”) in superconductivity, excitons in semiconductors, and phonons.
Examining emergent collective phenomena in terms of quasiparticles provided insight into a wide variety of physical settings, most notably in superconductivity and superfluidity, and recently in the famous example of Dirac quasiparticles in graphene. But so far, the observation and use of quasiparticles have been limited to quantum physics: in classical condensed matter, the collision rate is typically much too high to allow long-lived particle-like excitations.
However, The standard view that quasiparticles are exclusive to quantum matter has been recently challenged by a group of researchers at the Center for Soft and Living Matter (CSLM) within the Institute for Basic Science (IBS), South Korea. They examined a classical system made of microparticles driven by viscous flow in a thin microfluidic channel. As the particles are dragged by the flow, they perturb the streamlines around them, thereby exerting hydrodynamic forces on each other.
Remarkably, the researchers found that these long-range forces make the particles organize in pairs. This is because the hydrodynamic interaction breaks Newton’s third law, which states that the forces between two particles must be equal in magnitude and opposite in direction. Instead, the forces are ‘anti-Newtonian’ because they are equal and in the same direction, thus stabilizing the pair.
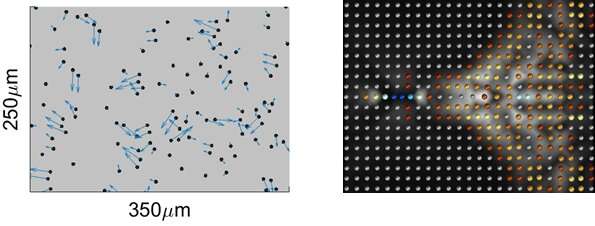
The large population of particles coupled in pairs hinted that these are the long-lived elementary excitations in the system—its quasiparticles. This hypothesis was proven right when the researchers simulated a large two-dimensional crystal made of thousands of particles and examined its motion. The hydrodynamic forces among the particles make the crystal vibrate, much like the thermal phonons in a vibrating solid body.
These pair quasiparticles propagate through the crystal, stimulating the creation of other pairs through a chain reaction. The quasiparticles travel faster than the speed of phonons, and thus every pair leaves behind an avalanche of newly-formed pairs, just like the Mach cone generated behind a supersonic jet plane. Finally, all those pairs collide with each other, eventually leading to the melting of the crystal.

The melting induced by pairs is observed in all crystal symmetries except for one particular case: the hexagonal crystal. Here, the three-fold symmetry of hydrodynamic interaction matches the crystalline symmetry and, as a result, the elementary excitations are extremely slow low-frequency phonons (and not pairs as usual). In the spectrum, one sees a “flat band” where these ultra-slow phonons condense. The interaction among the flat-band phonons is highly collective and correlated, which shows in the much sharper, different class of melting transition.
Notably, when analyzing the spectrum of the phonons, the researchers identified conical structures typical of Dirac quasiparticles, just like the structure found in the electronic spectrum of graphene. In the case of the hydrodynamic crystal, the Dirac quasiparticles are simply particle pairs, which form thanks to the ‘anti-Newtonian’ interaction mediated by the flow. This demonstrates that the system can serve as a classical analog of the particles discovered in graphene.
“The work is a first-of-its-kind demonstration that fundamental quantum matter concepts—particularly quasiparticles and flat bands—can help us understand the many-body physics of classical dissipative systems,” explains Tsvi Tlusty, one of the corresponding authors of the paper.
Moreover, quasiparticles and flat bands are of special interest in condensed matter physics. For example, flat bands were recently observed in double layers of graphene twisted by a specific “magic angle”, and the hydrodynamic system studied at the IBS CSLM happens to exhibit an analogous flat band in a much simpler 2D crystal.
“Altogether, these findings suggest that other emergent collective phenomena that have been so far measured only in quantum systems may be revealed in a variety of classical dissipative settings, such as active and living matter,” says Hyuk Kyu Pak, one of the corresponding authors of the paper.
More information: Imran Saeed, Quasiparticles, flat bands and the melting of hydrodynamic matter, Nature Physics (2023). DOI: 10.1038/s41567-022-01893-5. www.nature.com/articles/s41567-022-01893-5
Journal information: Nature Physics
Provided by Institute for Basic Science