by Michael W. Crowder, The Conversation
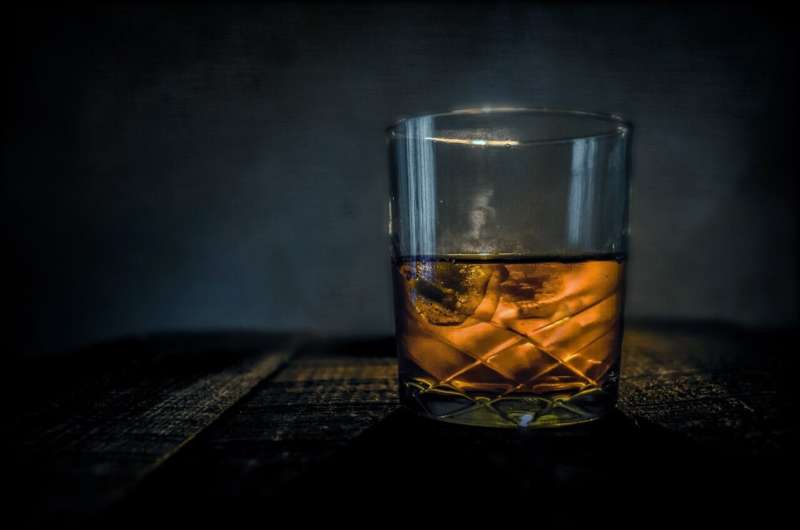
Few beverages have as rich a heritage and as complicated a chemistry as bourbon whiskey, often called “America’s spirit.” Known for its deep amber hue and robust flavors, bourbon has captured the hearts of enthusiasts across the country.
But for a whiskey to be called a bourbon, it has to adhere to very specific rules. For one, it needs to be made in the U.S. or a U.S. territory—although almost all is made in Kentucky. The other rules have more to do with the steps to make it—how much corn is in the grain mixture, the aging process and the alcohol proof.
I’m a bourbon researcher and chemistry professor who teaches classes on fermentation, and I’m a bourbon connoisseur myself. The complex science behind this aromatic beverage reveals why there are so many distinct bourbons, despite the strict rules around its manufacture.
The mash bill
All whiskeys have what’s called a mash bill. The mash bill refers to the recipe of grains that makes up the spirit’s flavor foundation. To be classified as bourbon, a spirit’s mash bill must have at least 51% corn—the corn gives it that characteristic sweetness.
Almost all bourbons also have malted barley, which lends a nutty, smoky flavor and provides enzymes that turn starches into sugars later in the production process.
Many distillers also use rye and wheat to flavor their bourbons. Rye makes the bourbon spicy, while wheat produces a softer, sweeter flavor. Others might use grains like rice or quinoa—but each grain chosen, and the amount of each, affects the flavor down the line.
The chemistry of yeast
Once distillers grind the grains from the mash bill and mix them with heated water, they add yeast to the mash. This process is called “pitching the yeast.” The yeast consumes sugars and produces ethyl alcohol and carbon dioxide as byproducts during the process called fermentation—that’s how the bourbon becomes alcoholic.
The fermented mash is now called “beer.” While similar in structure and taste to the beer you might buy in a six-pack, this product still has a way to go before it reaches its final form.
Yeast fermentation yields other byproducts besides alcohol and carbon dioxide, including flavor compounds called congeners. Congeners can be esters, which produce a fruity or floral flavor, or complex alcohols, which can taste strong and aromatic.
The longer the fermentation period, the longer the yeast has to create more flavorful byproducts, which enhances the complexity of the spirit’s final taste. And different yeasts produce different amounts of congeners.
Separating the fermentation products
During distillation, distillers separate the alcohol and congeners from the fermented mash of grains, resulting in a liquid spirit. To do this, they use pot or column stills, which are large kettles or columns, respectively, often made at least partially of copper. These stills heat the beer and any congeners that have a boiling point of less than 350°F (176°C) to form a vapor.
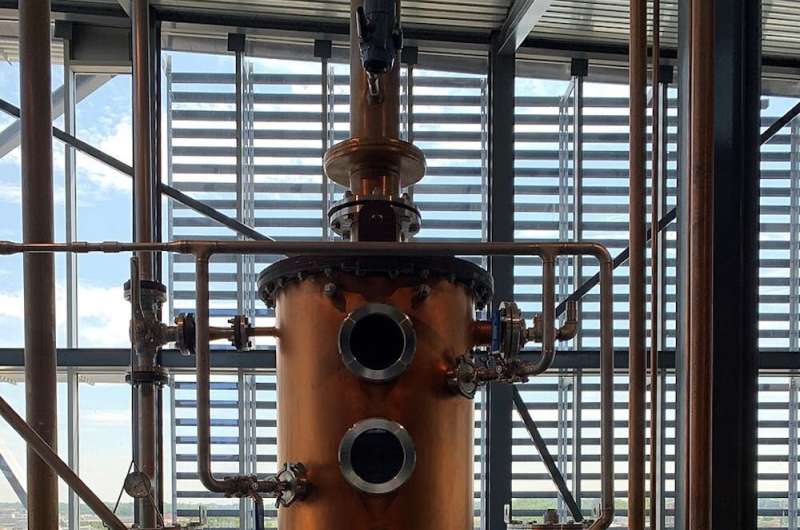
The type of still will influence the beverages’ final flavor, because pot stills often do not separate the congeners as precisely as column stills do. Pot stills result in a spirit that often contains a more complex mixture of congeners.
The desired vapors that exit the still are condensed back to liquid form, and this product is called the distillate.
Different chemical compounds have different boiling points, so distillers can separate the different chemicals by collecting the distillate at different temperatures. So in the case of the pot still, as the kettle is heated, chemicals that have lower boiling points are collected first. As the kettle heats further, chemicals with higher boiling points vaporize and then are condensed and collected.
By the end of the distillation process with a pot still, the distillate has been divided into a few fractions. One of these fractions is called the “hearts,” containing mostly ethanol and water, but also small amounts of congeners, which play a big role in the final flavor of the product.
The alchemy of time and wood
After distillation, the “hearts” fraction (which is clear and resembles water) is placed in a charred oak barrel for the aging process. Here, the bourbon interacts with chemicals in the barrel’s wood, and about 70% of the bourbon’s final flavor is determined by this step. The bourbon gets all its amber color during the aging process.
Bourbon may rest in the barrel for several years. During the summer, when the temperature is hot, the distillate can pass through the inner charred layer of the barrel. The charred wood acts like a filter and strains out some of the chemicals before the distillate seeps into the wood. These chemicals bind to the charred layer and do not release, kind of like a water filter.
Under the charred layer of the barrel is a “red line,” a layer where the oak was toasted during the charring process of making the barrel. The toasting process breaks down starch and other polymers, called lignins and tannins, in the oak.
When the distillate seeps to the red-line layer, it dissolves the sugars in the barrel, as well as lignin byproducts and tannins.
During the cold winter months, the distillate retreats back into the barrel, but it takes with it these sugars, tannins and lignin byproducts from the wood, which enhance the flavors. If you disassemble a barrel after it has aged bourbon, you can see a “solvent line,” which shows how far into the wood the distillate penetrated. The type of oak barrel can have a profound effect on the final taste, along with the barrel’s size and how charred it is.
For most distilleries, barrels are stored in large buildings called rickhouses. Ethyl alcohol and water in the distillate evaporate out of the barrel, and the humidity in that part of the rickhouse plays a big role.
Lower humidity often leads to higher-proof bourbon, as more water than ethanol leaves the barrel. In addition, air enters the barrel, and oxygen from the air reacts with some of the chemicals in the bourbon, creating new flavor chemicals. These reactions tend to soften the taste of the final product.
There are thousands of bourbons on the market, and they can be distinguished by their unique flavors and aromas. The variety of brands reflects the many choices that distillers make on the mash bill, fermentation and distillation conditions, and aging process. No two bourbons are quite the same.
Provided by The Conversation
This article is republished from The Conversation under a Creative Commons license. Read the original article.