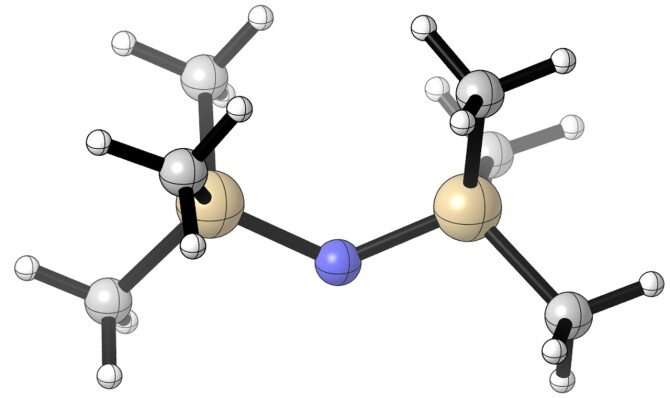
How to harness the potential of highly reactive radical molecules to work in pairs and spur transformative chemistry?
Bulk them up.
That is the approach of a new Cornell-led collaboration that attached large fragments to the infamously temperamental molecules, increasing their girth to insulate them from their hyperreactive partners.
The technique could prove to be a boon for creating new and improved derivatives of pharmaceutical compounds.
The group’s paper, “Regioselective Aliphatic C–H Functionalization Using Frustrated Radical Pairs,” published July 5 in Nature. The lead author is doctoral student Zhipeng Lu.
The project, led by Song Lin, professor of chemistry and chemical biology in the College of Arts and Sciences, emerged from the Lin Group’s previous experiments with synthetic electrochemistry. In that process, electrodes pass an electrical current through a chemical reaction to activate inert molecules that will form chemical bonds that otherwise might not be achievable.
Electrochemistry also happens to be one of the most efficient ways to generate high reactive radicals from simple chemical feedstocks.
“That’s where we thought, hey, when we have these radicals, how can we control them as well? If you can harness them and use them to react with a pharmaceutical, they can do really cool chemistry,” Lin said. “It’s really our interest in electrochemistry and radical chemistry that allowed us to think about these fundamental problems.”
Radicals are highly reactive, but they have the potential to bond in pairs by sharing a pair of single electrons. The challenge is getting them close enough to cooperate without annihilating each other.
The researchers’ solution was to bulk up the radicals by attaching groups of carbon and hydrogen atoms to their surface, effectively giving each molecule a set of antlers that allowed them to preserve their native reactivity while keeping their partner at a safe distance—also known as “frustrating” them.
“We use frustrated radicals to activate carbon-hydrogen bonds and convert them into other chemical bonds, which can affect the property of the original molecule,” Lin said. “This strategy can thus be used to improve efficacy of drug molecules, for example.”
Carbon-hydrogen bonds are ideal candidates for the task because they are commonly found in organic molecules—a carbon molecule, for example, can have upwards of 20 or 30 bonds. They are also quite strong, which is why they are often conscripted into service for pharmaceutical development.
At the same time, due to their strength, carbon-hydrogen bonds can be difficult to separate out. And because they are so plentiful, selectively functionalizing individual sites is not easy.
Lin’s group collaborated with researchers from the San Francisco-based company Genentech to identify substrate targets that would enable the desired chemical reactions. The team installed large functional groups, such as trimethylsilyl, on the frustrated radicals.
“You have this barrel of substituents to make it more hindered,” Lin said. “It is actually a very simple idea. How do you use size to control the reactivity and use them to do something useful?”
Once the products were made, the team teased them apart and analyzed the reactivity and selectivity through high-level nuclear magnetic resonance and gas and liquid chromatography.
The group’s technique can help medicinal chemists initiate dozens of different chemical transformations for a range of applications, from derivatizing more efficient pharmaceutical products and enhancing their biological activity to tracking how drugs degrade in the human body.
Co-authors include postdoctoral researchers Minsoo Ju and Yi Wang; recent Cornell graduate Jonathan Meinhardt; former postdoctoral researcher Jesus Martinez Alvarado; and researchers Elisia Villemure and Jack Terrett from Genentech.
More information: Zhipeng Lu et al, Regioselective aliphatic C–H functionalization using frustrated radical pairs, Nature (2023). DOI: 10.1038/s41586-023-06131-3
Journal information: Nature
Provided by Cornell University