by Chinese Academy of Sciences
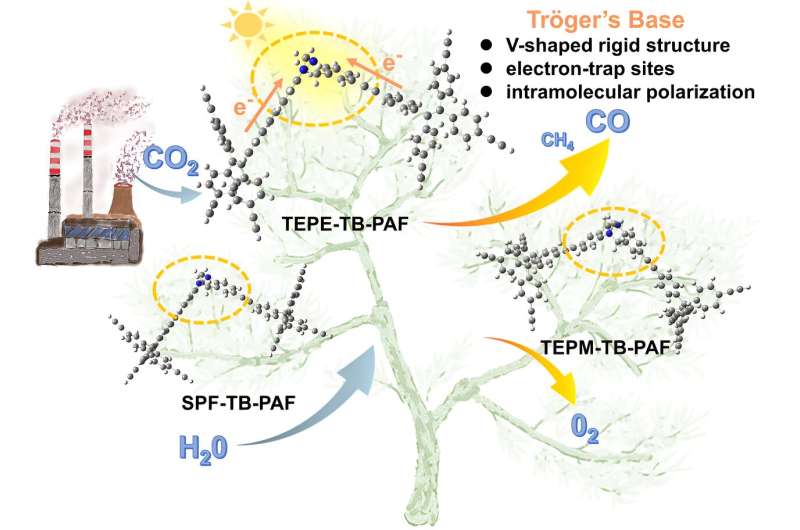
The global energy crisis is exacerbated by the continuous consumption of fossil fuels during the rapid development of modern industries. In addition, the survival and development of human beings is also being seriously affected by the emission of greenhouse gases.
As we know, natural photosynthesis can convert solar illumination to sustainable energy. Inspired by this, a carbon-neutral strategy that uses sunlight to convert carbon dioxide to fuels has attracted great attention.
The successful implementation of photosynthesis depends on the design of photocatalysts. Inorganic semiconductors have been extensively studied for CO2 photoreduction reaction (CO2PRR), which presents shortcomings. For example, the band gaps of metal oxides and metal chalcogenides are difficult to adjust, resulting in poor light absorption. In addition, the high carrier recombination rate and the low Brunauer-Emmett-Teller (BET) specific surface area impair the photocatalytic efficiency, thus limiting photon utilization.
Photocatalysts based on porous organic frameworks (POFs) have attracted tremendous interest owing to their facile bandgap and charge separation tunability via designable functional building blocks. Nevertheless, a considerable portion of previously reported POFs still rely on the metal reactive sites. The photosensitizer and sacrificial reagent are required for promoting their performances on CO2PRR.
These are obviously uneconomical. The photoreduction of CO2 tends to produce mixed products with different ratios as the number of electrons and protons reacting with CO2 varies. The quantity and purity of the targeted product are generally low due to insufficient conversion, which requires overcoming the high chemical inertness of CO2. Therefore, achieving highly efficient and selective CO2 conversion have been very challenging.
A research team led by Prof. Yong Yang from Nanjing University of Science and Technology and Prof. Yong Zhou from Nanjing University, China, reported a series of three-dimensional Tröger’s base-derived porous aromatic frameworks (3D-X-TB-PAFs (X = TEPE, TEPM, SPF)) featuring designated reaction sites and unique charge transfer properties. The results were published in Chinese Journal of Catalysis.
The incorporation of V-shaped Tröger’s base (TB) units and aromatic alkynes both endow polymers with permanent porosity, additional photon scattering cross-sections and enhanced CO2 adsorption/activation capability. Both density functional theory (DFT) calculations and optoelectronic measurements reveal the formation of intramolecular built-in polarization and electron-trap sites brought about by TB, which modulate the charge separation and customize reaction sites in collaboration with the enhanced CO2 accumulation.
Also, the product allocation in the photoreduction of CO2 is hereby regulated together with the photooxidation of H2O. Among the 3D-PAFs, the most efficient electron transport channel is constructed in TEPE-TB-PAF with the full conjugated TEPE-T.
In the absence of cocatalysts and sacrificial agents, TEPE-TB-PAF presents a competitive CO formation rate (194.50 μmol g-1 h-1) with near-unity selectivity (99.74%). Significantly, the low energy barrier for CO desorption and high energy barrier for *CHO formation commonly contribute to the high efficiency of TEPE-TB-PAF, which is demonstrated by computational explorations and in-situ diffuse reflectance infrared Fourier transform (DRIFT) spectra.
This work provides efficient building blocks for the synthesis of multifunctional organic photocatalysts and a ground-breaking insight for the simultaneous enhancement of photocatalytic reactivity and selectivity.
More information: Nan Yin et al, Tröger’s base derived 3D-porous aromatic frameworks with efficient exciton dissociation and well-defined reactive site for near-unity selectivity of CO2 photo-conversion, Chinese Journal of Catalysis (2023). DOI: 10.1016/S1872-2067(23)64474-2
Provided by Chinese Academy of Sciences