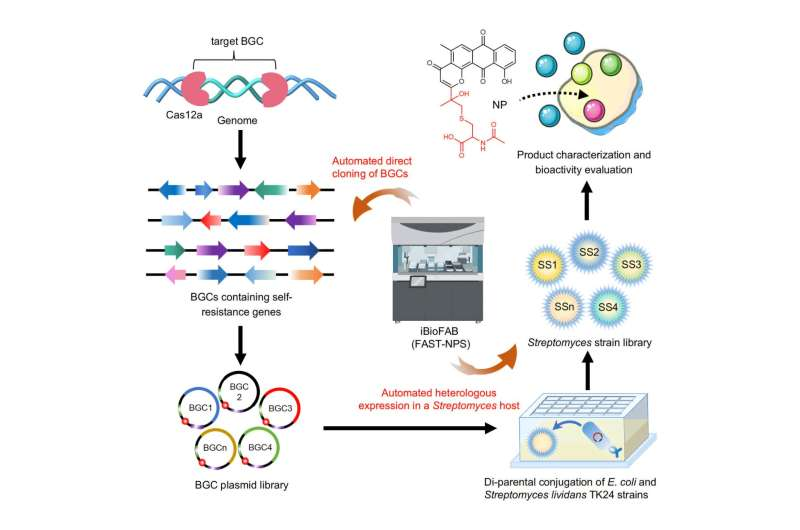
From caffeine to penicillin, natural products have become a mainstay in modern society, and are used for numerous applications, such as medicine and pesticides. There are tens of thousands of untapped natural products, but identifying new compounds with bioactivity can be difficult, especially when produced in low quantities.
To overcome this, researchers at the University of Illinois Urbana-Champaign have developed FAST-NPS, a new method to accelerate the discovery and scale up of bioactive natural products found in Streptomyces. The work is published in the journal Cell Systems.
Natural products are chemicals produced by living organisms that are either essential for the organism to survive or provide secondary functions such as protection from infection or predators. The discovery of natural products derived from bacteria, fungi, and plants has been particularly advantageous for the development of lifesaving medicines, including antibiotics like erythromycin, daptomycin, and vancomycin, which have been isolated from Streptomyces.
With advancements in computational technology and genomic sequencing, researchers can use microbial genome mining to more efficiently discover new natural products, compared to traditional methods that require isolation directly from organisms. But there are still improvements that can be made to these processes, because not all natural products demonstrate bioactive properties and many are produced in low quantities, making them hard to detect.
Natural products are structurally diverse, coming in a variety of shapes and sizes that can often be too complex for chemists to synthesize. However, nature proves to be the best chemist, using numerous enzymes to build these natural products in microorganisms. The genes that encode the required enzymes are often located together in the genome to form so-called biosynthetic gene clusters, BGCs. Using bioinformatics and machine learning techniques, researchers can identify BGCs in microbial genomes to discover new natural products.
“In this work, we try to address two challenges in natural product discovery. The first challenge is how we can efficiently identify new compounds that are bioactive,” said Huimin Zhao, the Steven L. Miller Chair of Chemical and Biomolecular Engineering (BSD theme leader/CABBI/CGD/MMG). “The second challenge is how we can scale up our CAPTURE method using our robotic system.”
To tackle the first challenge, the research study, led by postdoctoral researcher and first author of the paper Yujie Yuan, leveraged self-resistance genes.
“We are using the self-resistance genes as markers to prioritize BGCs of natural products with bioactivity,” Yuan said.
Self-resistance genes are an evolutionary response to shield organisms from any negative effects that could be caused by their own natural products. So the self-resistance genes in the BGCs serve as a predictor of the bioactivity of the corresponding natural products, therefore improving the efficiency of the discovery process by narrowing the pool of BGCs to further pursue and test.
After identifying a target BGC using the Antibiotic Resistant Target Seeker tool, ARTS, its genes are captured from the microbial genome and inserted into bacteria that can synthesize the natural product. Zhao’s group had previously reported a high-efficiency direct cloning method coupled with heterologous expression to accomplish this.
“In the past, we developed the CAPTURE method, which allows us to clone large biosynthetic gene clusters from microbial genomes with high efficiency. Although the process is very efficient, it is tedious and involves a lot of manual work,” Zhao said.
The team adapted their CAPTURE and heterologous expression methods into a fully automated, scalable, high-throughput platform, called FAST-NPS, for discovering bioactive natural products in Streptomyces. FAST-NPS integrates seamlessly with the ARTS tool.
By fully automating this process using the Illinois Biological Foundry for Advanced Biomanufacturing, iBioFAB, they are able to eliminate this tedious manual labor and increase the number of BCGs they can pursue in parallel. In a process that takes almost a month from start to finish, automation increases the capability to clone and express BGCs from about ten at a time to several hundred at a time.
“We spent a lot of time and effort to develop the automation workflow. This was a big challenge because we had to develop each component, from PCR and RNA transcription to bacterial transformation and heterologous expression,” Yuan said. “I am very proud of our CAPTURE method and am excited to report this more powerful version for the discovery of bioactive compounds.”
The team’s efforts paid off because the proof-of-concept demonstrated a 95% success rate when they cloned 105 BGCs from 11 Streptomyces strains. Further, they identified five promising BGCs, and all five proved to produce bioactive compounds.
But moving forward, Zhao says that there is still room for improvement. “While we had a 100% success rate for discovering bioactive compounds, five is a small number because we still need to improve the success rate of the heterologous expression. We cloned more than 100 biosynthetic gene clusters, and only 12 were functionally expressed. So, this is a challenge that we will try to address.”
More information: Yujie Yuan et al, Self-resistance-gene-guided, high-throughput automated genome mining of bioactive natural products from Streptomyces, Cell Systems (2025). DOI: 10.1016/j.cels.2025.101237
Journal information: Cell Systems
Provided by University of Illinois at Urbana-Champaign