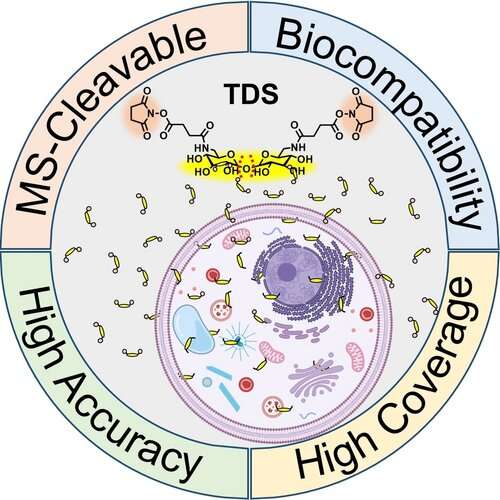
As the executor of life activities, proteins exert their specific biological functions through interactions such as forming protein complexes. The localization effects, crowding effects, and organelle microenvironments within cells are crucial for maintaining the structure and function of protein complexes.
Recently, a research team led by Prof. Zhang Lihua from the Dalian Institute of Chemical Physics (DICP) of the Chinese Academy of Sciences (CAS) has developed a glycosidic-bond-based mass-spectrometry-cleavable cross-linker, which improves the data analysis throughput and identification accuracy of cross-linking information with good amphiphilicity and biocompatibility. It enables in-vivo cross-linking of protein complexes in live cells and achieves large-scale and precise analysis. The study was published in Angewandte Chemie International Edition on March 30.
Chemical cross-linking mass spectrometry (CXMS), especially in-vivo CXMS, is a large-scale analysis of in-situ conformation and interaction interface of protein complexes in living cells. However, in-vivo CXMS in living cells faces challenges such as high cell disturbance and complex spectra retrieval of cross-linked peptides.
In this study, the researchers incorporated glycosidic bonds into the design of functional cross-linkers based on the high biocompatibility of glucose molecules and the mass spectrometry cleavable feature of glycosidic bonds. They screened and obtained trehalose, a highly biocompatible molecule, as the skeleton molecule and developed a mass spectrometry cleavable cross-linker, trehalose disuccinimidyl succinate (TDS).
This cross-linker showed superior cell viability maintenance compared to currently reported membrane-permeable chemical cross-linkers and enabled efficient cross-linking of protein complexes in cells under low disturbance conditions.
The researchers found that low-energy glycosidic bond–high-energy peptide bond mass spectrometry selective fragmentation mode reduced analysis complexity of the cross-linked peptide fragment spectra, significantly improving the efficiency and accuracy of cross-linked peptide identification.
They identified conformation of 1,453 proteins corresponding to more than 3,500 cross-linked peptide pairs, and 843 protein-protein interaction information from Hela cells.
“We have accurately realized in-vivo cross-linking and global analysis of protein complexes in live cells, and provided an important toolkit for exploring the interaction sites of protein function regulation in live cell microenvironment,” said Prof. Zhang.
More information: Jing Chen et al, A Glycosidic‐Bond‐Based Mass‐Spectrometry‐Cleavable Cross‐linker Enables In Vivo Cross‐linking for Protein Complex Analysis, Angewandte Chemie International Edition (2023). DOI: 10.1002/anie.202212860
Journal information: Angewandte Chemie International Edition
Provided by Chinese Academy of Sciences