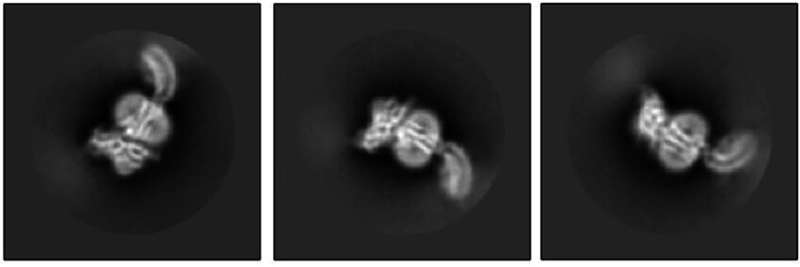
As the proverb goes, it takes a village to raise a child. It can also take a village to make progress in science and medicine.
In this case, labs from not one, not two, but three departments in the Blavatnik Institute at Harvard Medical School, along with a colleague in France, came together to figure out the structure of a tricky receptor involved in heart, lung, liver, and kidney function as well as pregnancy.
Untangling this structure, described April 20 in Nature Chemical Biology, provides a foundation for developing drugs that act on the receptor with the goal of treating heart disease and conditions marked by the buildup of scar tissue, or fibrosis. Those include idiopathic pulmonary fibrosis, a chronic disease of the lungs; non-alcoholic fatty liver disease; and scleroderma, which affects the skin, joints, and internal organs.
The work may also pique the interest of other scientists who study basic biology because the receptor has an unusual structure that stands out from other members of its family, the G protein-coupled receptors.
“This was a very difficult receptor to characterize,” said senior author Andrew Kruse, HMS professor of biological chemistry and molecular pharmacology. “The collaboration of multiple labs was essential, and the project highlights the value of combining experimental and computational methods.”
“You have a lot of great opportunities at HMS to work with talented scientists who use different techniques than you,” agreed Sarah Erlandson, who led the work as a graduate student in the Kruse lab. “That allowed us to form a more complete picture of the receptor and study it in more ways than we could by ourselves.”
A relaxin possibility
The receptor’s name is a mouthful: relaxin/insulin-like family peptide receptor 1, or RXFP1. It gets that name because it and its three receptor siblings bind to hormones called relaxins.
Relaxins are best known for initiating a constellation of changes in the body during pregnancy, including relaxing ligaments in the pelvis in preparation for childbirth. It also boosts sperm movement. But scientists have grown to appreciate the hormone’s many non-reproductive roles as well, including dilating blood vessels to increase blood flow, stimulating the growth of new blood vessels, breaking down collagen, and reducing inflammation and fibrosis.
Relaxins do all of this when they get released from tissues such as the heart, prostate, and placenta and bind to receptors in the membranes of cells in certain organs. The receptors then send signals that spur the cells to act.
Most relaxins stay local, but one type, relaxin-2, travels throughout the body via the blood. This is the relaxin that binds to RXFP1.
Relaxin-2’s involvement in so many bodily processes has turned scientists’ eyes toward treating diseases by mimicking higher or lower levels of the hormone. Doing so requires designing drugs that bind to RXFP1—which is hard to do without knowing its structure.
“There are no drugs available that target this receptor,” said Erlandson, who now works as a research scientist at Takeda Pharmaceuticals. “People are interested in it as an option for treatment of cardiovascular and fibrotic diseases, but when you don’t understand the detailed structure, it limits your ability to target it.”
So, the teams got to work.
It takes two to tango, four to solve a receptor structure
The Kruse lab took the first step by using cryo-electron microscopy to reveal what RXFP1 looks like at the near-atomic level when bound to relaxin-2.
But one blurry spot remained where a flexible part of the receptor kept changing position from one snapshot to the next. That elusive part was the most important one—the part that binds to relaxin.
Members of the lab of Steven Gygi, HMS professor of cell biology, tackled the problem using mass spectrometry, a different method for determining structural information that measures atomic weights. Combining the experimental cryo-EM results with the mass spectrometry data allowed Erlandson to fill in the missing structural details.
Now the researchers could see the receptor in its active, or “on,” state, bound to relaxin-2. The structure suggested that the receptor could turn itself on. If that were true, what prevented it from being on all the time, sending cell-activation signals whether relaxin was there or not?
Insight came from Debora Marks, HMS associate professor of systems biology, and Xiaojing Cong at the Institute of Functional Genomics (IGF) in France. They used computational techniques—including one called evolutionary coupling analysis, which looks at protein sequences that change together over time—to predict how different parts of the receptor might shift around between its active and inactive states.
At last, the story revealed itself.
When RXFP1 is alone, no relaxins in sight, it’s turned off. When relaxin-2 binds to it, multiple parts of the receptor change shape and communicate with one another to flip the “on” switch.
The collaboration allowed the team to answer open questions about how RXFP1’s multiple parts move around and work together to allow the receptor to do its job. The way it binds to relaxin-2 hasn’t been seen in many of its receptor relatives.
With RXFP1’s active structure in hand, researchers now have a lock to design therapeutic keys for.
“It was really exciting to get to the point in the project where we saw the structure and were making these discoveries,” said Erlandson. “There’s more work to be done, but this makes a big contribution that benefits scientists and could ultimately help patients.”
Additional authors are Shaun Rawson, James Osei-Owusu, Kelly P. Brock, Xinyue Liu, Joao A. Paulo, and Julian Mintseris, of HMS.
More information: Sarah C. Erlandson et al, The relaxin receptor RXFP1 signals through a mechanism of autoinhibition, Nature Chemical Biology (2023). DOI: 10.1038/s41589-023-01321-6
Journal information: Nature Chemical Biology
Provided by Harvard Medical School