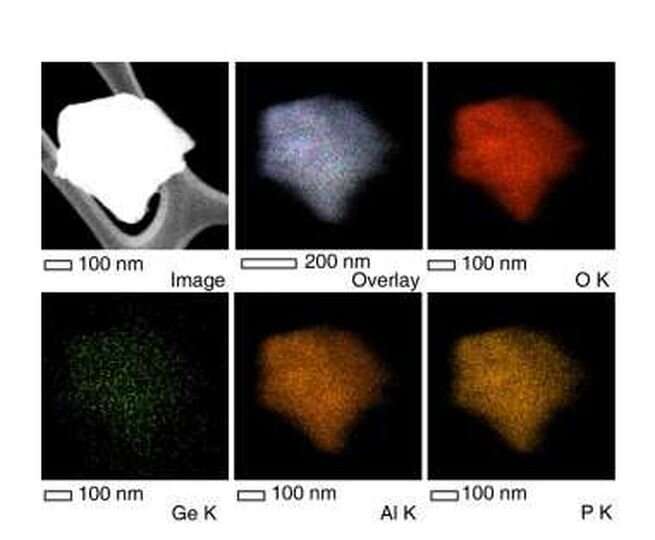
Researchers have reported a strategy to disentangle the activity-selectivity tradeoff for direct conversion of syngas, a mixture of carbon monoxide and hydrogen, into desirable ethylene, propylene, and butylene. These hydrocarbons are known as light olefins and are the most-used building blocks for plastics.
“Activity and selectivity are two primary indexes of a successful catalyst for chemical reactions. A higher activity means higher efficiency in converting feedstock to products, thereby reducing energy consumption,” said Jiao Feng, an associate professor at the Dalian Institute of Chemical Physics at the Chinese Academy of Sciences in Dalian, China. “Selectivity reflects the percentage of the desired products; for example, ethylene, propylene and butylene in this case, which determines the economy of the technology.”
For almost a century, a process called the Fischer-Tropsch synthesis (FTS) was used for direct syngas conversion with iron or cobalt-based catalysts for synthesis of chemicals. However, selectivity for light olefins remained a challenge. An alternative process, named OXZEO and developed six years ago by the same research team using metal oxide-zeolite catalyst, improved light-olefin selectivity far beyond the theoretical limit of FTS. Despite the significant progress over the years, the activity is still limited by the activity-selectivity tradeoff.
For example, when FTS is used to convert syngas to light olefins, the yield amounts to around 26%. Using traditional silicon containing zeotypes within the OXZEO catalyst concept, the light-olefins yield has so far maxed out at 27%. These limits originate from activity-selectivity tradeoff, a long-standing challenge in catalysis. This can be traced to the catalytic sites for both the target and side reactions, which are usually entangled on technical catalysts.
Now, in a paper published in the journal Science on May, 18, 2023, a team led by Dr. Jiao, Prof. Pan and Prof. Bao has shown that incorporating germanium-substituted aluminophosphates within the OXZEO catalyst concept can disentangle the desired target reaction from the undesired secondary reactions. It enhances the conversion of the intermediates to produce olefins by creating more active sites and in turn generation of intermediates but without degrading the selectivity of light olefins. With this new strategy, researchers simultaneously achieved high CO conversion and light-olefins selectivity and the yield reached an unprecedented 48% under optimized conditions.
To validate the mechanism, researchers also studied silicon-substitute and magnesium-substitute aluminophosphates and tested them in similar scenarios. The active sites of these two zeotypes cannot efficiently shield the side reaction of hydrogenation and oligomerization, thereby the activity-selectivity tradeoff cannot be overcome, despite optimizing the acid site density or reaction conditions.
“Separating the active sites of the two key steps of syngas conversion via OXZEO catalysts, and increasing the active site density and modulating its properties for kinetics of intermediate transport and reactions within the zeotype confined pores provides one effective solution for syngas conversion to light olefins,” said Pan Xiulian, professor at the Dalian Institute of Chemical Physics at the Chinese Academy of Sciences in Dalian, China. “We expect that this can be applicable to analogous bifunctional catalysis in other reactions and will be of interest for further development of zeolite catalysis.”
“If it can be incorporated with green hydrogen energy technology in the future, it will make significant contribution to the goal of carbon neutrality,” said Bao Xinhe, professor at the Dalian Institute of Chemical Physics at the Chinese Academy of Sciences in Dalian and the President of the University of Science and Technology of China.
More information: Feng Jiao et al, Disentangling the activity-selectivity tradeoff in catalytic conversion of syngas to light olefins, Science (2023). DOI: 10.1126/science.adg2491
Journal information: Science
Provided by Chinese Academy of Sciences