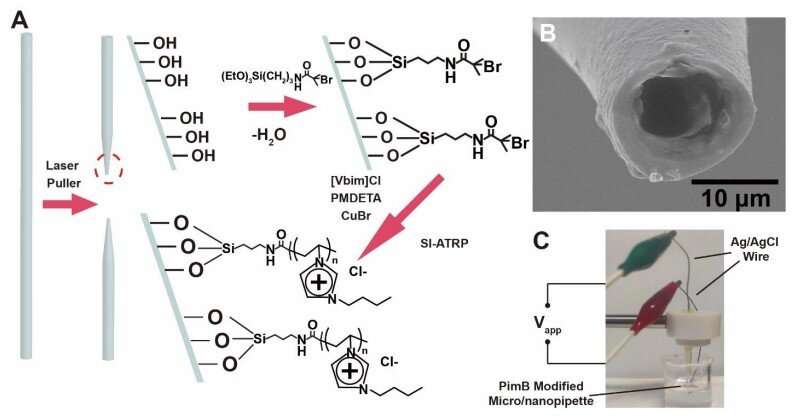
Neuromorphic devices have attracted increasing attention because of their potential applications in neuromorphic computing, intelligence sensing, brain-machine interfaces and neuroprosthetics. However, most of the neuromorphic functions realized are based on the mimic of electric pulses with solid state devices. Mimicking the functions of chemical synapses, especially neurotransmitter-related functions, is still a challenge in this research area.
In a study published in Science, the research group led by Prof. Yu Ping and Mao Lanqun from the Institute of Chemistry of the Chinese Academy of Sciences developed a polyelectrolyte-confined fluidic memristor (PFM), which could emulate a diverse electric pulse with ultralow energy consumption. Moreover, benefitting from the fluidic nature of PFM, chemical-regulated electric pulses and chemical-electric signal transduction could also be emulated.
The researchers first fabricated the polyelectrolyte-confined fluidic channel by surface-initiated atomic transfer polymerization. By systematically studying the current-voltage relationship, they found that the fabricated fluidic channel could be identified as a memristor, defined as PFM. The origin of the ion memory came from the relatively slow diffusion dynamics of anions into and out of the polyelectrolyte brushes.
The PFM could emulate short-term plasticity patterns (STP), including paired-pulse facilitation and paired-pulse depression. These functions can be operated at a voltage and energy consumption as low as those biological systems, suggesting the potential application in bioinspired sensorimotor implementation, intelligent sensing and neuroprosthetics.
The PFM could also emulate the chemical-regulated STP electric pulses. Based on the interaction between polyelectrolyte and counterions, the retention time could be regulated in different electrolytes. More importantly, in a physiological electrolyte (i.e., phosphate-buffered saline solution, pH 7.4), the PFM could emulate the regulation of memory by adenosine triphosphate (ATP), demonstrating the possibility to regulate the synaptic plasticity by neurotransmitter.
Based on the interaction between polyelectrolytes and counterions, the chemical-electric signal transduction was accomplished with the PFM, which is a key step towards the fabrication of artificial chemical synapses.
With structural emulation to ion channels, PFM features versatility and easily interfaces with biological systems, paving a way to building neuromorphic devices with advanced functions by introducing rich chemical designs. This study provides a new way to interface chemistry with neuromorphic devices.
More information: Tianyi Xiong et al, Neuromorphic functions with a polyelectrolyte-confined fluidic memristor, Science (2023). DOI: 10.1126/science.adc9150
Journal information: Science
Provided by Chinese Academy of Sciences