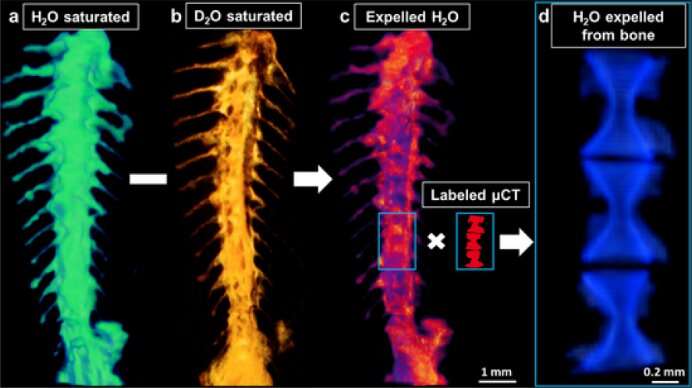
Around 500 million years ago, early vertebrates in the seas became fish, adopting an inner skeleton and a flexible spine based on a nanocomposite of fibers and mineral, known as bone material. This “invention” of evolution was so successful that the basic structure was also adopted for later vertebrates that lived on land.
However, while the bones of all terrestrial vertebrates are basically equipped with bone cells (osteocytes), certain fish species continued to evolve and finally managed to create a more energy efficient material: bone lacking bone cells, found today for example in fish such as salmon, medaka or tilapia.
Samples with and without bone cells
“We asked ourselves how bone samples with and without bone cells actually differ in their microstructures and properties,” says Prof. Paul Zaslansky, who heads a research group at Charité Berlin and specializes in mineralized biomaterials including teeth and bones.
Together with Ph.D. student Andreia Silvera and international partners, they have now compared bone samples from zebrafish and medaka. Both fish species are of similar size and live in similar conditions, so their skeletons must withstand similar stresses. However, while zebrafish have bone cells, the skeleton of medaka do not.
“The background to the question is that the function of bone cells in bone and how they change with age is of great interest to the aging population,” Silvera explains. Bone cells can respond to physical stress by sending biochemical signals that lead to the formation or resorption of bone tissue, adapting to load. But with age or in diseases such as osteoporosis, this mechanism no longer seems to work.
“With our basic research, we want to find out how bones with and without bone cells differ and cope with the challenges of external stress,” Zaslansky says.
Strength and elasticity
Bones have a complex structure: they comprise nanofibers of collagen and nanoparticles of mineral but also other minor ingredients. Certain protein compounds, so called Proteoglycans (PGs), are embedded in a tissue of collagen fibers and nanocrystals and play important roles in tissue formation and maintenance.
“PGs may be compared to salt in the soup. Too little or too much of it is not good,” Zaslansky says. The PGs can retain water, and there are plenty of PGs in healthy cartilage, making it as elastic as a sponge. Together, these components form an extracellular matrix (ECM), a 3D structure that provides strength and elasticity, ensuring function for many years.
In bones, an open network (Lacunar Channel Network or LCN) of channels and pores with diameters ranging from a few hundred nanometers to micrometers is created in this 3D structure. This LCN hosts the bone osteocytes, cells that sense load and orchestrate bone remodeling. In the LCN and within the nanocomposite, bone contains up to 20% of its volume in water, with many functions including toughening and adaptation to mechanical stress.
Neutron tomography at BER II
To determine the amount of incorporated water, the researchers first immersed bone samples in water and transilluminated them with neutrons, provided by the Berlin experimental reactor BER II at HZB—followed by saturation in deuterated heavy water (D2O). 3D data was collected again and the difference between the two bone states allowed the team to determine for each spine vertebrae the precise amount of water displaced by diffusion of the D2O.
“In addition, we examined sections of the bone samples, analyzed them by electron microscopy and micro CT and we also determined the PG concentration with Raman spectroscopy,” Silvera explains.
Surprising results: PGs make the difference
Until now, it was assumed that both bone types contain similar amounts of water and had very similar composition and properties. In fact however, the neutron examination showed that the bone material of zebrafish releases half as much water as that of medaka. This is all the more surprising because these bones have a very similar microstructure of mineralized collagen fibers, but zebrafish also contain large cell spaces within the LCN.
“My first reaction was, ‘This must be wrong!’ So we checked everything thoroughly and realized it’s really revolutionary,” recalls Zaslansky. The only explanation for the difference is that the bone matrices of the two species differ in a fundamental compositional component that affects water permeability. And here, both histological studies and Raman spectroscopy show: it’s the small but important contribution of PGs. The medaka samples contain far less PG than the zebrafish samples.
“This is a new finding: although both fish cope with similar stresses, their bone materials do not have the same water permeability properties,” says Silveira.
The study is published in the journal Materials & Design.
“We hope these results will help us better understand bone diseases as well,” Zaslansky says. Why are some bones better at responding to stress than others? What happens when bones age? Could it be that they lose PGs, and become less watertight? Perhaps aging or pathology such as osteoporosis changes the bone that surrounds bone cells, which makes it difficult to remodel and form bone tissue that works correctly?
More information: Andreia Silveira et al, Water flow through bone: Neutron tomography reveals differences in water permeability between osteocytic and anosteocytic bone material, Materials & Design (2022). DOI: 10.1016/j.matdes.2022.111275
Provided by Helmholtz Association of German Research Centres