by Zhang Nannan, Chinese Academy of Sciences
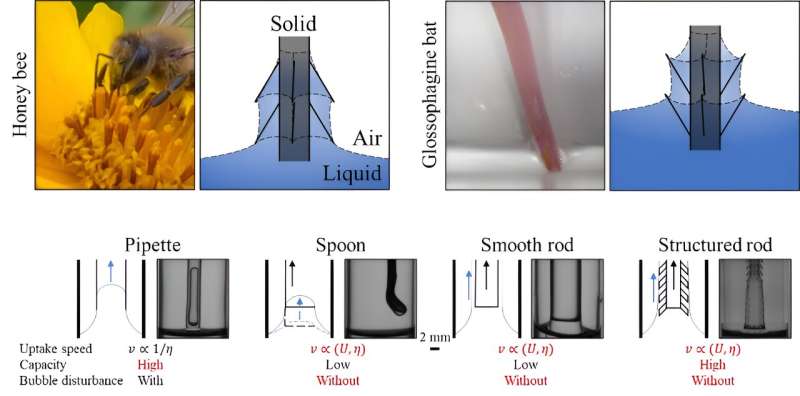
In a study published in Science Advances, Chinese researchers have demonstrated the dynamics of liquid entrainment on solid surfaces with millimeter structures.
In some industrial processes, when transferring viscous liquid from a confined space, common tools such as pipettes and spoons are limited. The pipette is limited by high viscosity and low vacuum level. It also easily generates annoying bubbles during suction or pressure release. The confined space limits the spoon’s ability to pick up liquid from below.
For honey bees and glossophagine bats, viscous nectar is entrained and ingested as their long, hairy tongues dip into and out of the nectar. Common intuition would suggest that viscosity is undesirable in general fluid transfer processes. In this example, however, higher viscosity ensures higher, not lower, entrainment as the “hairs” on the tongue, measured in millimeters or submillimeters, further promote entrainment.
Inspired by this phenomenon, researchers from the Technical Institute of Physics and Chemistry (TIPC) of the Chinese Academy of Sciences, and the Suzhou Institute for Advanced Research of the University of Science and Technology of China integrated two key processes of liquid entrainment applied in industry, i.e., “liquid uptake” and “liquid drainage” after uptake, and found that millimeter structures moderately improve the overall entrainment process.
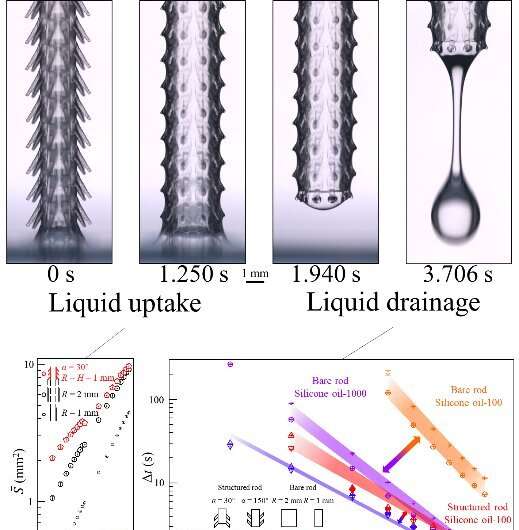
The essence of the entrainment process is a solid architecture passing through the interface of two fluids. For common liquids and typical industrial settings, millimeter scale is where the force such as gravity, the capillary effect, and the viscous force have the same magnitude. The viscous and capillary effects provide the driving force for entrainment, while gravity acts as the resistance. These forces control the fluid behavior and modify the classical Landau-Levich-Derjaguin theory of entrainment. Therefore, millimetric structures can accommodate liquid in larger quantities and with less deviation.
Meanwhile, liquid drainage from a millimeter-structured surface is distinct from easy drainage from an unstructured surface or the firmly trapped state of a micro-structured surface. In an uptake-drainage process, the amount of uptake is increased, which lowers friction and promotes dripping. This occurs even though higher viscosity tends to increase friction and inhibit dripping. These two opposite effects reach a good balance at the millimeter scale. The capillary force further reduces the relative influence of the viscous force. As a result, millimeter structures achieve relatively uniform droplet dripping time across a range of viscosities.
Enhanced uptake and uniform drainage combine for unique superiority in liquid transfer. The researchers built a modularized apparatus with a programmable multi-axis displacement table holding a detachable array of millimeter-structured rods. The apparatus cyclically transferred viscous liquid, much like a conveyor belt in an industrial plant. The liquid was drawn from narrow centrifuge tubes and dripped down at a convenient frequency for one to operate in practical applications. Because of the uniform draining effect, this apparatus and its settings could be adapted to liquids of varying viscosity.
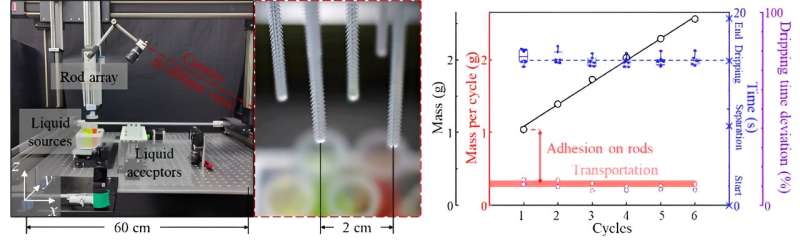
As a brief demonstration, photosensitive resin was then transferred. The resin droplets were quickly cured under ultraviolet light in the short time interval between two transfer cycles. This demonstration showed that batch fabrication, packaging, or modification of lenses, chips, or other similar devices could be achieved through this process.
Uneven surfaces of millimeter morphology are ubiquitous: They include toys being painted, nasopharyngeal swabs for virus testing, tires running through a puddle, and intestinal villi absorbing nutrients, among other things. Relative motion often occurs between these surfaces and the liquid droplet, stream, bath, or water body. For this reason, an understanding of liquid entrainment is required to better control processes involving such uneven surfaces.
More information: Ziyang Cheng et al, Viscous-capillary entrainment on bioinspired millimetric structure for sustained liquid transfer, Science Advances (2023). DOI: 10.1126/sciadv.adi5990
Journal information: Science Advances
Provided by Chinese Academy of Sciences