by Tejasri Gururaj , Phys.org
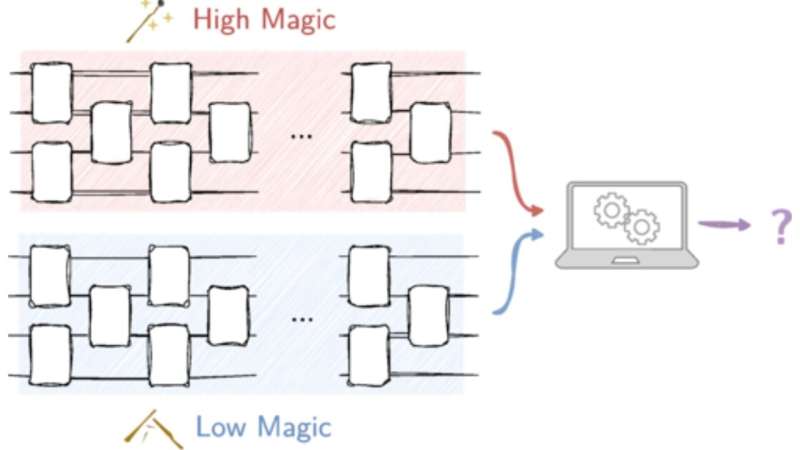
A new study in Physical Review Letters (PRL) introduces the concept of pseudomagic quantum states, which appear to have high stabilizerness (or complexity) and can move us closer to achieving quantum supremacy.
Quantum supremacy or quantum advantage is the ability of quantum computers to simulate or run computations that classical computers can’t (due to their limited computational abilities).
Achieving universal quantum computation is the ability of quantum computers to be able to perform any arbitrary quantum computation, and quantum supremacy is at the heart of this.
The new PRL study explores nonstabilizer states or magic states. These are quantum states that allow quantum computations that cannot be efficiently simulated on classical computers. This complexity is what gives quantum computers their potential power.
Phys.org spoke to co-authors of the paper Andi Gu, a Ph.D. student at Harvard University, and Dr. Lorenzo Leone, a postdoctoral researcher at Freie Universität, Berlin.
“The starting point to understand our research is that quantum computation is more powerful than classical computation. In quantum computing, the term nonstabilizerness or magic refers to a measure of the non-classical resources possessed by a quantum state,” explained Gu.
Stabilizer vs. nonstabilizer quantum states
Every quantum system can be represented as a quantum state, a mathematical equation containing all the information about the system.
A stabilizer state is a type of quantum state that can be efficiently simulated (or executed) on a classical computer.
“These states—along with a restricted set of quantum operations called stabilizer operations—form a classically simulable framework. However, stabilizer states and operations alone are not sufficient for achieving universal quantum computation,” explained Dr. Leone.
To perform computations that are truly quantum and beyond classical capabilities, nonstabilizer states are required. These states can enable quantum computers to perform tasks infeasible for classical computers. However, one of the main challenges is constructing these magic states.
Nonstabilizer states are inherently challenging to construct as they require more complex quantum operations.
“In this context, nonstabilizerness is best viewed as a resource because it is essential for achieving quantum advantage. The more nonstabilizerness a quantum state possesses, the more powerful it is as a resource for quantum computation,” explained Gu.
Pseudomagic states
The researchers found a way around this challenge by introducing the concept of pseudomagic quantum states.
Pseudomagic quantum states appear to have the properties of nonstabilizer states (complexity and non-classical operations) but are computationally indistinguishable from random quantum states, at least to an observer with limited computational resources.
In simple terms, this means that pseudomagic quantum states appear like magic states but are far less complex to construct. Especially to someone with a not-so-powerful computer, pseudomagic quantum states are indistinguishable from random quantum states.
“This indistinguishability arises from the fact that efficiently distinguishing between pseudomagic states and truly magical states would require an exponential amount of computational resources, making it infeasible for any realistic observer,” said Dr. Leone.
Gu added, “Just as pseudorandom number generators produce sequences that appear random to computationally limited classical observers, pseudomagic states are engineered to appear highly nonstabilizer to computationally bounded quantum observers.”
Laying down the foundations
Over the course of six theorems, the researchers laid out the theoretical foundation for pseudomagic states as well as their implications for quantum computing applications.
They constructed the pseudomagic states in a way that the gap between their actual and apparent nonstabilizerness was tunable.
“This means that we can create states that may seem to be powerful resources for quantum computation, even though they are not as resource-intensive as they appear,” explained Dr. Leone.
The core of this framework revolved around the concept of stabilizer entropy. This is a measure of the nonstabilizerness (or complexity) of a quantum system.
What is unique about the stabilizer entropy is that, unlike other measurements of nonstabilizerness, it is computationally less draining.
Implications for quantum computing applications
The researchers focused on three areas where pseudomagic states could have implications, beginning with quantum cryptography.
According to the study, pseudomagic states introduce a new protocol for quantum cryptography based on EFI (or Efficiently preparable, statistically Far, but computationally Indistinguishable) pairs.
These pairs can improve the security of data communication and can be constructed using pseudomagic states.
The researchers also show that pseudomagic states can provide new insights into quantum chaos and scrambling, which are important for understanding the behavior of complex quantum systems and the spread of quantum information.
“By demonstrating that the apparent magic of a quantum state can differ from its actual magic, our work highlights the need to consider the limitations of realistic, computationally limited observers when studying quantum systems and their applications,” explained Gu.
Finally, they also demonstrate that pseudomagic states can be used to build more efficient fault-tolerant quantum computers using a process called magic state distillation.
Magic state distillation is essentially a purification process that improves the fidelity of the magic states, making them more suitable for use in quantum algorithms and error-correction schemes.
The researchers wish to explore the relationship between pseudomagic states and concepts in quantum information theory in the future. Additionally, they want to explore the experimental realization of pseudomagic states with existing and near-term quantum devices.
“This could lead to the development of practical applications that harness the unique properties of these states,” concluded Dr. Leone.
by Tejasri Gururaj