![Reconnecting current sheets and magnetic flux ropes in MHD turbulence. (A) Volume rendering of the current density ∣J∣ in the entire domain at a stage when turbulence is fully developed. Myriad of current sheets is evident in the plane perpendicular to the mean magnetic field B z0 . (B) and (C) depict one reconnecting current sheet and the embedded flux ropes in a small subdomain [within the boundaries ( − 0.45, − 0.4) × (0.45, 0.5) × (0.95, 1.05)]. (B) shows the volume rendering of ∣J∣, while (C) displays magnetic field lines (colored by ∣B∣) associated with the featured current sheet (including magnetic flux ropes) and an x-y slice view of the current density component, J z , along the mean magnetic field. (D) Out-of-plane current density J z in an x-y slice (at z = −1) of the 3D turbulence simulation (left) compared with the corresponding result from a 2D simulation (right). Copious formation of magnetic flux ropes/plasmoids occurs in both 3D and 2D simulations despite the different morphology. Zoomed-in subdomains are used to illustrate the increased morphological complexity that characterizes the 3D simulation. Credit: Science Advances (2022). DOI: 10.1126/sciadv.abn7627 The world's largest turbulence simulation unmasks the flow of energy in astrophysical plasmas](https://scx1.b-cdn.net/csz/news/800a/2022/the-worlds-largest-tur-1.jpg)
Researchers have uncovered a previously hidden heating process that helps explain how the atmosphere that surrounds the sun called the “solar corona” can be vastly hotter than the solar surface that emits it.
The discovery at the U.S. Department of Energy’s (DOE) Princeton Plasma Physics Laboratory (PPPL) could improve tackling a range of astrophysical puzzles such as star formation, the origin of large-scale magnetic fields in the universe, and the ability to predict eruptive space weather events that can disrupt cell phone service and black out power grids on Earth. Understanding the heating process also has implications for fusion research.
First clear 3D explanation
“Our direct numerical simulation is the first to provide clear identification of this heating mechanism in 3D space,” said Chuanfei Dong, a physicist at PPPL and Princeton University who unmasked the process by conducting 200 million hours of computer time for the world’s largest simulation of its kind. “Current telescope and spacecraft instruments may not have high enough resolution to identify the process occurring at small scales,” said Dong, who details the breakthrough in the journal Science Advances.
The hidden ingredient is a process called magnetic reconnection that separates and violently reconnects magnetic fields in plasma, the soup of electrons and atomic nuclei that forms the solar atmosphere. Dong’s simulation revealed how rapid reconnection of the magnetic field lines turns the large-scale turbulent energy into small-sale internal energy. As a consequence the turbulent energy is efficiently converted to thermal energy at small scales, thus superheating the corona.
“Think of putting cream in coffee,” Dong said. “The drops of cream soon become whorls and slender curls. Similarly, magnetic fields form thin sheets of electric current that break up due to magnetic reconnection. This process facilitates the energy cascade from large-scale to small-scale, making the process more efficient in the turbulent solar corona than previously thought.”
When the reconnection process is slow while the turbulent cascade is fast, reconnection cannot affect the transfer of energy across scales, he said. But when the reconnection rate becomes fast enough to exceed the traditional cascade rate, reconnection can move the cascade toward small scales more efficiently.
It does this by breaking and rejoining the magnetic field lines to generate chains of small twisted lines called plasmoids. This changes the understanding of the turbulent energy cascade that has been widely accepted for more than half a century, the paper says. The new finding ties the energy transfer rate to how fast the plasmoids grow, enhancing the transfer of energy from large to small scales and strongly heating the corona at these scales.
The new discovery demonstrates a regime with an unprecedentedly large magnetic Reynolds number as in the solar corona. The large number characterizes the new high energy transfer rate of the turbulent cascade. “The higher the magnetic Reynolds number is, the more efficient the reconnection-driven energy transfer is,” said Dong, who is moving to Boston University to take up a faculty position.
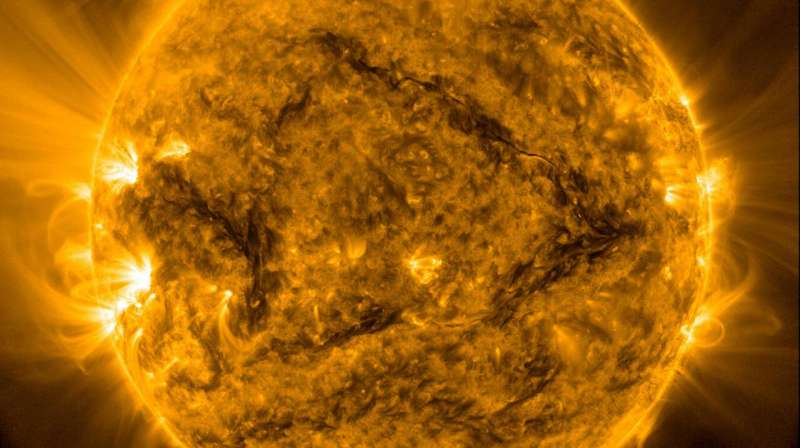
200 million hours
“Chuanfei has carried out the world’s largest turbulence simulation of its kind that has taken over 200 million computer CPUs [central processing units] at the NASA Advanced Supercomputing (NAS) facility,” said PPPL physicist Amitava Bhattacharjee, a Princeton professor of astrophysical sciences who supervised the research. “This numerical experiment has produced undisputed evidence for the first time of a theoretically predicted mechanism for a previously undiscovered range of turbulent energy cascade controlled by the growth of the plasmoids.
“His paper in the high-impact journal Science Advances completes the computational program he began with his earlier 2D results published in Physical Review Letters. These papers form a coda to the impressive work that Chuanfei has done as a member of the Princeton Center for Heliophysics, a joint Princeton and PPPL facility. We are grateful for a PPPL LDRD [Laboratory Directed Research & Development] grant that facilitated this work, and to the NASA High-End Computing (HEC) program for its generous allocation of computer time.”
The impact of this finding in astrophysical systems across a range of scales can be explored with current and future spacecraft and telescopes. Unpacking the energy transfer process across scales will be crucial to solving key cosmic mysteries, the paper said.
More information: Chuanfei Dong et al, Reconnection-driven energy cascade in magnetohydrodynamic turbulence, Science Advances (2022). DOI: 10.1126/sciadv.abn7627
Journal information: Physical Review Letters , Science Advances
Provided by Princeton Plasma Physics Laboratory